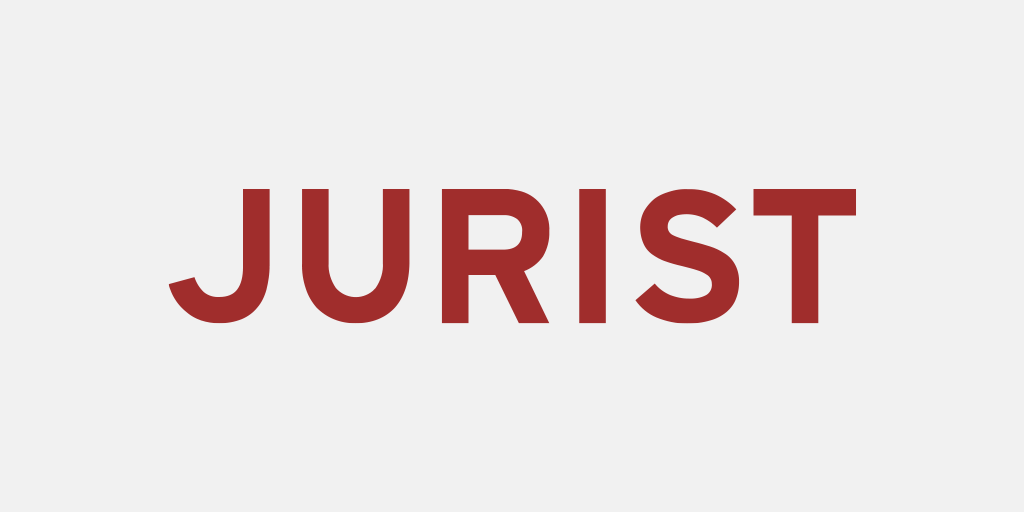
Table of Contents Back to Top
- DATES:
- ADDRESSES:
- FOR FURTHER INFORMATION CONTACT:
- SUPPLEMENTARY INFORMATION:
- Background
- Previous Federal Actions
- Species Information
- Taxonomy and Species Description
- Distribution
- Distribution in the Conterminous United States
- Native Distribution of Arctic Grayling in the Upper Missouri River Basin
- Introduced Lake-Dwelling Arctic Grayling in the Upper Missouri River Basin
- Origins, Biogeography, and Genetics of Arctic Grayling in North America
- Habitat
- Breeding
- General Life History Diversity
- Life History Diversity in Arctic Grayling in the Upper Missouri River Basin
- Age and Growth
- Distinct Vertebrate Population Segment
- Discreteness
- Significance
- Unique Ecological Setting
- Gap in the Range
- Only Surviving Natural Occurrence of the Taxon That May Be More Abundant Elsewhere as an Introduced Population Outside of Its Historical Range
- Differs Markedly in Its Genetic Characteristics
- Allozymes
- Mitochondrial DNA
- Microsatellite DNA
- Phenotypic Characteristics Influenced by Genetics—Meristics
- Inference Concerning Genetic Differences in Arctic Grayling of the Missouri River Relative to Other Examples of the Taxon
- Determination of Distinct Population Segment
- Population Status and Trends of Populations in the Upper Missouri River DPS
- Distinct Population Segment Five-Factor Analysis
- Summary of Information Pertaining to the Five Factors
- Factor A. The Present or Threatened Destruction, Modification, or Curtailment of Its Habitat or Range
- Curtailment of Range and Distribution
- Dams on Mainstem Rivers
- Water Management in the Upper Missouri River Basin
- Habitat Fragmentation/Smaller Seasonal Barriers
- Degradation of Riparian Habitat
- Dewatering From Irrigation and Consequent Increased Water Temperatures
- Entrainment
- Sedimentation
- Climate Change
- Conservation Efforts To Reduce Habitat Destruction, Modification, or Curtailment of Its Range
- Big Hole River CCAA
- Conservation Efforts by Landowners Not Enrolled in the CCAA
- Big Hole River Drought Management Plan
- Native Arctic Grayling Genetic Reserves and Translocation
- Summary of Factor A
- B. Overutilization for Commercial, Recreational, Scientific, or Educational Purposes
- Recreational Angling
- Monitoring and Scientific Study
- Reintroduction Efforts
- Conservation Efforts To Reduce Recreational Overutilization
- Summary of Factor B
- Factor C. Disease or Predation
- Disease
- Predation By and Competition With Nonnative Trout
- Predation by Birds and Mammals
- Summary of Factor C
- Factor D. The Inadequacy of Existing Regulatory Mechanisms
- U.S. Federal Laws and Regulations
- National Environmental Policy Act
- Federal Land Policy and Management Act
- National Forest Management Act
- National Park Service (NPS) Organic Act
- National Wildlife Refuge System Improvement Act of 1997
- Federal Power Act (FPA)
- Clean Water Act
- State Laws
- Montana Environmental Policy Act
- Laws Affecting Physical Aquatic Habitats
- Montana Water Use Act
- Angling Regulations
- Summary of Factor D
- Factor E. Other Natural or Manmade Factors Affecting Its Continued Existence
- Drought
- Stochastic (Random) Threats, Genetic Diversity and Small Population Size
- Summary of Factor E
- Cumulative Effects From Factors A Through E
- Climate Change and Nonnative Species Interactions
- Climate Change and Dewatering
- Summary
- Finding
- Significant Portion of the Range
- References Cited
- Authors
- Authority
Tables Back to Top
- Table 1—Geographic Distribution, Genetic Status, and Source of Introduced Adfluvial Arctic Grayling Populations in the Upper Missouri River Basin
- Table 2—Differences Between the Ecological Setting of the Upper Missouri River and Elsewhere in the Species’ Range of Arctic Grayling
- Table 4—Conservation Projects and Results, and Arctic Grayling Response in the Big Hole River Since Implementation of the Big Hole CCAA in 2006
- Table 5—Comparison of Number of Days Varying Flow Targets Were Achieved Among Similar Years of Below Average Snowpack in the Big Hole River CCAA Management Segment C, Pre- and Post CCAA. All Information in This Table Cited From Roberts 2014, Unpublished Data
DATES: Back to Top
The finding announced in this document was made on August 20, 2014.
ADDRESSES: Back to Top
This finding is available on the Internet at http://www.regulations.gov at Docket Number FWS-R6-ES-2013-0120. Supporting documentation we used in preparing this finding is available for public inspection, by appointment, during normal business hours at the U.S. Fish and Wildlife Service, Montana Ecological Services Office, 585 Shepard Way, Suite 1, Helena, MT 59601. Please submit any new information, materials, comments, or questions concerning this finding to the above street address.
FOR FURTHER INFORMATION CONTACT: Back to Top
Jodi Bush, Field Supervisor, Montana Ecological Services Office (see ADDRESSES); telephone 406-449-5225. If you use a telecommunications device for the deaf (TDD), please call the Federal Information Relay Service (FIRS) at 800-877-8339.
SUPPLEMENTARY INFORMATION: Back to Top
Background Back to Top
Section 4(b)(3)(B) of the Act (16 U.S.C. 1531 et seq.) requires that, for any petition to revise the Federal Lists of Endangered and Threatened Wildlife and Plants that contains substantial scientific or commercial information that listing the species may be warranted, we make a finding within 12 months of the date of receipt of the petition. In this finding, we will determine that the petitioned action is: (1) Not warranted, (2) warranted, or (3) warranted, but the immediate proposal of a regulation implementing the petitioned action is precluded by other pending proposals to determine whether species are endangered or threatened, and expeditious progress is being made to add or remove qualified species from the Federal Lists of Endangered and Threatened Wildlife and Plants. We must publish these 12-month findings in the Federal Register.
Previous Federal Actions
We have published a number of documents on Arctic grayling since 1982, and have been involved in litigation over previous findings. We describe previous federal actions that are relevant to this document below.
We published our first status review for the Montana Arctic grayling (Thymallus arcticus montanus), then thought to be a subspecies of Arctic grayling, in a Federal Register document on December 30, 1982 (47 FR 58454). In that document, we designated the purported subspecies, Montana Arctic grayling, as a Category 2 species. At that time, we designated a species as Category 2 if a listing as endangered or threatened was possibly appropriate, but we did not have sufficient data to support a proposed rule to list the species.
On October 9, 1991, the Biodiversity Legal Foundation and George Wuerthner petitioned us to list the fluvial (riverine) populations of Arctic grayling in the Upper Missouri River basin as an endangered species throughout its historical range in the coterminous United States. We published a notice of a 90-day finding in the January 19, 1993, Federal Register (58 FR 4975), concluding the petitioners presented substantial information indicating that listing the fluvial Arctic grayling of the Upper Missouri River in Montana and northwestern Wyoming may be warranted. This finding also noted that taxonomic recognition of the Montana Arctic grayling (Thymallus arcticus montanus) as a subspecies (previously designated as a category 2 species) was not widely accepted, and that the scientific community generally considered this population a geographically isolated member of the wider species (T. arcticus).
On July 25, 1994, we published notification of a 12-month finding in the Federal Register (59 FR 37738), concluding that listing the DPS of fluvial Arctic grayling in the Upper Missouri River was warranted but precluded by other higher priority listing actions. This DPS determination predated our DPS policy (61 FR 4722, February 7, 1996), so the entity did not undergo a DPS analysis as described in the policy. The 1994 finding placed fluvial Arctic grayling of the Upper Missouri River on the candidate list and assigned it a listing priority of 9, indicating that the threats were imminent but of moderate to low magnitude.
On May 31, 2003, the Center for Biological Diversity and Western Watersheds Project (Plaintiffs) filed a complaint in U.S. District Court in Washington, DC, challenging our 1994 “warranted but precluded” determination for the DPS of fluvial Arctic grayling in the Upper Missouri River basin. On May 4, 2004, we elevated the listing priority number of the fluvial Arctic grayling to 3 (69 FR 24881), indicating threats that were imminent and of high magnitude. On July 22, 2004, the Plaintiffs amended their complaint to challenge our failure to emergency list this population. We settled with the Plaintiffs in August 2005, and we agreed to submit a revised determination on whether this population warranted listing as endangered or threatened to the Federal Register on or before April 16, 2007.
On April 24, 2007, we published a revised 12-month finding on the petition to list the Upper Missouri River DPS of fluvial Arctic grayling (72 FR 20305) (“2007 finding”). In this finding, we determined that fluvial Arctic grayling of the upper Missouri River did not constitute a species, subspecies, or DPS under the Act. Therefore, we found that the upper Missouri River population of fluvial Arctic grayling was not a listable entity under the Act, and, as a result, listing was not warranted. With that document, we withdrew the fluvial Arctic grayling from our candidate list.
On November 15, 2007, the Center for Biological Diversity, Federation of Fly Fishers, Western Watersheds Project, George Wuerthner, and Pat Munday filed a complaint (CV-07-152, in the District Court of Montana) to challenge our 2007 finding. We settled this litigation on October 5, 2009. In the stipulated settlement, we agreed to: (a) Publish, on or before December 31, 2009, a document in the Federal Register soliciting information on the status of the upper Missouri River Arctic grayling; and (b) submit, on or before August 30, 2010, a new 12-month finding for the upper Missouri River Arctic grayling to the Federal Register.
On October 28, 2009, we published in the Federal Register a notice of intent to conduct a status review of Arctic grayling (Thymallus arcticus) in the upper Missouri River system (74 FR 55524). To ensure the status review was based on the best available scientific and commercial data, we requested information on the taxonomy, biology, ecology, genetics, and population status of the Arctic grayling of the upper Missouri River system; information relevant to consideration of the potential DPS status of Arctic grayling of the upper Missouri River system; threats to the species; and conservation actions being implemented to reduce those threats in the upper Missouri River system. That document further specified that the status review might consider various DPS designations that include different life histories of Arctic grayling in the upper Missouri River system and different DPS configurations, including fluvial, adfluvial (lake populations), or all life histories of Arctic grayling in the upper Missouri River system.
On September 8, 2010, we published a revised 12-month finding on the petition to list the Upper Missouri River DPS of Arctic grayling (75 FR 54708) (“2010 finding”). In this finding, we determined that fluvial and adfluvial Arctic grayling of the upper Missouri River did constitute a DPS under the Act. Further, we found that a DPS configuration including both adfluvial and fluvial life histories was the most appropriate for the long-term conservation of Arctic grayling because genetic evidence indicated that fluvial and adfluvial life-history forms did not represent distinct evolutionary lineages. We concluded by finding that the Upper Missouri River DPS of Arctic grayling was warranted for listing under the Act, but precluded by other higher priority listing actions.
On September 9, 2011, we reached an agreement with plaintiffs in Endangered Species Act Section 4 Deadline Litig., Misc. Action No. 10-377 (EGS), MDL Docket No. 2165 (D. D.C.) (known as the “MDL case”) on a schedule to publish proposed listing rules or not-warranted findings for the species on our candidate list. This agreement stipulated that we would submit for publication in the Federal Register either a proposed listing rule for the Upper Missouri River DPS of Arctic grayling, or a not-warranted finding, no later than the end of Fiscal Year 2014.
On November 26, 2013, we published a document in the Federal Register (78 FR 70525) notifying the public that we were initiating a status review of the Upper Missouri River DPS of Arctic grayling to determine whether the entity meets the definition of an endangered or threatened species under the Act. That document requested general information (taxonomy, biology, ecology, genetics, and status) on the Arctic grayling of the upper Missouri River system, as well as information on the conservation status of, threats to, planned and ongoing conservation actions for, habitat selection of, habitat requirements of, and considerations concerning the possible designation of critical habitat for the Arctic grayling of the upper Missouri River system.
This document constitutes a revised 12-month finding (“2014 finding”) on whether to list the Upper Missouri River DPS of Arctic grayling (Thymallus arcticus) as endangered or threatened under the Act, and fulfills our commitments under the MDL case.
Species Information
Taxonomy and Species Description
The Arctic grayling (Thymallus arcticus) is a fish belonging to the family Salmonidae (salmon, trout, charr, whitefishes), subfamily Thymallinae (graylings), and it is represented by a single genus, Thymallus. Arctic grayling have elongate, laterally compressed, trout-like bodies with deeply forked tails, and adults typically average 300-380 millimeters (mm) (12-15 inches (in.)) in length. Coloration can be striking, and varies from silvery or iridescent blue and lavender, to dark blue (Behnke 2002, pp. 327-328). A prominent morphological feature of Arctic grayling is the sail-like dorsal fin, which is large and vividly colored with rows of orange to bright green spots, and often has an orange border (Behnke 2002, pp. 327-328).
For more detail on taxonomy and species description, see the 2010 finding (75 FR 54708).
Distribution
Arctic grayling are native to Arctic Ocean drainages of Alaska and northwestern Canada, as far east as Hudson’s Bay, and westward across northern Eurasia to the Ural Mountains (Scott and Crossman 1998, pp. 301-302; Froufe et al. 2005, pp. 106-107; Weiss et al. 2006, pp. 511-512). In North America, they are native to northern Pacific Ocean drainages as far south as the Stikine River in British Columbia (Nelson and Paetz 1991, pp. 253-256; Behnke 2002, pp. 327-331).
For a full discussion on the global distribution of Arctic grayling, see the 2010 finding (75 FR 54709-54710). Here, we focus on the distribution of Arctic grayling within the conterminous United States.
Distribution in the Conterminous United States
Two disjunct groups of Arctic grayling were native to the conterminous United States: One in the upper Missouri River basin in Montana and Wyoming (currently extant only in Montana); and another in Michigan that was extirpated in the late 1930s (Hubbs and Lagler 1949, p. 44), and has not been detected since.
During the status review process, the Service received information indicating that Arctic grayling may have also been native to areas outside the Upper Missouri River basin in Montana and Wyoming. This information included multiple historical newspaper clippings and several reports from early Army expeditions purporting that Arctic grayling were captured in the Yellowstone River drainage in Montana and the Snake River drainage in Idaho (Shea 2014, entire). Some of these reports even included descriptions of captured fish. However, none of the descriptions mentions the colorful, sail-like dorsal fin of Arctic grayling, a prominent feature that clearly distinguishes Arctic grayling from other salmonids. In addition, a similar species resembling Arctic grayling (i.e., mountain whitefish) is native to both the Yellowstone River drainage and Snake River drainage. Mountain whitefish were sometimes referred to as “grayling” in some areas of the West (Ellis 1914, p. 75). Thus, it is likely that early reports of Arctic grayling occurring outside the upper Missouri River basin were mountain whitefish misidentified as Arctic grayling. Therefore, without information to the contrary, we consider Arctic grayling to be native only to the upper Missouri River basin in Montana and Wyoming and to Michigan.
Native Distribution of Arctic Grayling in the Upper Missouri River Basin
The first Euro-American “discovery” of Arctic grayling in North America is attributed to members of the Lewis and Clark Expedition, who encountered the species in the Beaverhead River in August 1805 (Nell and Taylor 1996, p. 133). Vincent (1962, p. 11) and Kaya (1992, pp. 47-51) synthesized accounts of Arctic grayling occurrence and abundance from historical surveys and contemporary monitoring to determine the historical distribution of the species in the upper Missouri River system (Figure 1). We base our conclusions on the historical distribution of Arctic grayling in the upper Missouri River basin on these two reviews. Arctic grayling were widely but irregularly distributed in the upper Missouri River system above the Great Falls in Montana and in northwest Wyoming within the present-day location of Yellowstone National Park (Vincent 1962, p. 11). They were estimated to inhabit up to 2,000 kilometers (km) (1,250 miles (mi)) of stream habitat until the early 20th century (Kaya 1992, pp. 47-51). Arctic grayling were reported in the mainstem Missouri River, as well as in the Smith, Sun, Jefferson, Madison, Gallatin, Big Hole, Beaverhead, and Red Rock Rivers (Vincent 1962, p. 11; Kaya 1992, pp. 47-51; USFWS 2007; 72 FR 20307, April 24, 2007). Anecdotal accounts report that the species may have been present in the Ruby River, at least seasonally (Magee 2005, pers. comm.), and were observed there as recently as the early 1970s (Holton, undated).
BILLING CODE 4310-55-P
BILLING CODE 4310-55-C
Fluvial Arctic grayling were historically widely distributed in the upper Missouri River basin, but a few adfluvial populations also were native to the basin. For example, Arctic grayling are native to Red Rock Lakes, in the Centennial Valley (Vincent 1962, pp. 112-121; Kaya 1992, p. 47). Vincent (1962, p. 120) stated that Red Rock Lakes were the only natural lakes in the upper Missouri River basin accessible to colonization by Arctic grayling, and concluded that Arctic grayling there were the only native adfluvial population in the basin. However, Arctic grayling were also native to Elk Lake (in the Centennial Valley; Kaya 1990, p. 44) and a few small lakes in the upper Big Hole River drainage, based on recent genetic information (Peterson and Ardren 2009, p. 1768).
The distribution of native Arctic grayling in the upper Missouri River went through a dramatic reduction in the first 50 years of the 20th century, especially in riverine habitats (Vincent 1962, pp. 86-90, 97-122, 127-129; Kaya 1992, pp. 47-53). The native populations that formerly resided in the Smith, Sun, Jefferson, Beaverhead, Gallatin, and mainstem Missouri Rivers are considered extirpated, and the only remaining native fluvial population is found in the Big Hole River and some of its tributaries (Kaya 1992, pp. 51-53). The fluvial form currently occupies less than 10 percent of its historical range in the Missouri River system (Kaya 1992, p. 51). Other native populations in the upper Missouri River occur in two small, headwater lakes in the upper Big Hole River system (Miner and Mussigbrod Lakes); the upper Ruby River (recently reintroduced from Big Hole River stock); the Madison River upstream from Ennis Reservoir; Elk Lake in the Centennial Valley (recently reintroduced from Red Rock Lakes stock); and the Red Rock Lakes in the Centennial Valley (Everett 1986, p. 7; Kaya 1992, p. 53; Peterson and Ardren 2009, pp. 1762, 1768; see Figure 1).
Introduced Lake-Dwelling Arctic Grayling in the Upper Missouri River Basin
From 1898 through the 1960s, an estimated 100 million Arctic grayling were stocked across Montana and other western States. The sources of these stockings varied through time as different State, Federal, and private hatchery operations were created, but the ultimate source for all hatcheries in Montana appears to be stock from two Montana populations: Centennial Valley and Madison River (Peterson and Ardren 2009, p. 1767; Leary 2014, unpublished data; MFISH 2014a). Arctic grayling derived from these two sources were stocked on top of every known native Arctic grayling population in the upper Missouri River basin. In addition, Arctic grayling were stocked in multiple high elevation lakes, some of which likely were historically fishless.
There are 20 known, introduced Arctic grayling populations that exist in the upper Missouri River basin. These 20 populations, along with the 6 populations existing in native habitat, comprise the listable entity (total of 26 populations) of Arctic grayling in the upper Missouri River basin. However, six of these introduced populations are considered to have low conservation value because they occupy unnatural habitat, are not self-sustaining, or are used as captive brood reserves. These six populations are Axolotl Lake, Green Hollow Lake, Sunnyslope Canal, Tunnel Lake, South Fork Sun River, and Elk Lake. The Axolotl and Green Hollow populations are captive brood reserves maintained in natural lakes for reintroduction purposes. Sunnyslope Canal is a fluvial population that occurs in unnatural habitat (irrigation canal). Tunnel Lake is stocked with “rescued” fish from Sunnyslope Canal, but lacks a spawning tributary and is consequently not self-sustaining (SSA 2014). South Fork Sun River is a small fluvial population that resides in about1/4mile of stream during the summer and is not considered self-sustaining (SSA 2014). The Elk Lake population is a genetic replicate of the Centennial Valley population, but no documented spawning has occurred to date (Jaeger 2014a, pers. comm.); thus this population is not currently considered self-sustaining. For these reasons, we primarily focus our analysis on the populations considered to have high conservation value; those populations that are self-sustaining, in natural habitats, and wild.
The 14 known remaining introduced, lake-dwelling (adfluvial) Arctic grayling populations within the upper Missouri River basin are likely the result of historical stocking (Table 1). In our 2010 finding, we considered and discussed the conservation value of these populations. Based on the information available at that time, we considered these introduced populations to not have conservation value for multiple reasons. Below, we list each of the reasons for this conclusion as provided in the 2010 finding, and provide an updated assessment and conclusion about the potential conservation value of these populations, based on new information obtained since 2010.
Population | Drainage | Genetic analysis completed? | Sourcea | Citation |
---|---|---|---|---|
aOrigin of source stock was determined by genetic analysis and through analysis of historical stocking records and scientific literature, in some cases. Where multiple sources are cited, fish from each population were known to be stocked, although the genetic contribution of each donor population to the current population structure is unknown. | ||||
bThese populations are the result of reintroductions using known sources of Montana origin. | ||||
cSchwinegar and Park Lakes Arctic grayling populations are likely from Montana-origin sources due to proximity to other lakes with known Montana origin; however, definitive evidence is lacking. | ||||
Agnes Lake | Big Hole | No | Madison/Centennial | MFISH 2014a. |
Odell Lake | Big Hole | Yes | Centennial | Peterson and Ardren 2009, p. 1766; Leary 2014, unpublished data. |
Bobcat Lake | Big Hole | Yes | Centennial | Peterson and Ardren 2009, p. 1766; Leary 2014, unpublished data. |
Schwinegar Lake | Big Hole | No | Madison/Centennial.c | |
Pintlar Lake | Big Hole | Yes | Madison/Centennial | Leary 2014, unpublished data. |
Deer Lake | Gallatin | Yes | Madison/Centennial | Leary 2014, unpublished data. |
Emerald Lake | Gallatin | Yes | Madison/Centennial | Leary 2014, unpublished data. |
Grayling Lake | Gallatin | Yes | Madison/Centennial | Leary 2014, unpublished data. |
Hyalite Lake | Gallatin | Yes | Madison/Centennial | Leary 2014, unpublished data. |
Diversion Lake | Sun | Yesb | Big Hole | Horton 2014a, pers. comm.; Magee 2014, pers. comm. |
Gibson Reservoir | Sun | Yesb | Big Hole | Horton 2014a, pers. comm.; Magee 2014, pers. comm. |
Lake Levale | Sun | Yesb | Big Hole | Horton 2014a, pers. comm.; Magee 2014, pers. comm. |
Park Lake | Missouri | No | Madison/Centennial.c | |
Grebe Lake | Madison | Yes | Centennial | Peterson and Ardren 2009, p. 1766; Varley 1981, p. 11. |
1. The Service interprets the Act to provide a statutory directive to conserve species in their native ecosystems (49 FR 33885, August 27, 1984) and to conserve genetic resources and biodiversity over a representative portion of a taxon’s historical occurrence (61 FR 4722, February 7, 1996). Since most of the introduced populations of Arctic grayling were of unknown genetic origin and in lakes that were likely historically fishless, these populations were considered in 2010 to be outside the species’ native range, and we concluded that they did not appear to add conservation value to the DPS.
Since 2010, new genetic information from 7 of the 14 introduced populations indicates there are moderate to high levels of genetic diversity within and among these populations, and indicates these populations were derived from native sources within the upper Missouri River basin (Leary 2014, unpublished data; Table 1). In addition, stocking records show common stocking sources for introduced populations that were genotyped (as described previously) and the two populations that were not genotyped (the remaining 3 populations were reintroductions of known Montana origin sources; Table 1). Thus, it appears that all 14 introduced Arctic grayling populations contain moderate to high levels of genetic diversity of Arctic grayling in the upper Missouri River basin that was not captured within the DPS designation in the 2010 finding.
The Service’s current interpretation of the Act is consistent with that in the 2010 finding; we believe it is important to conserve species in their native ecosystems and to conserve genetic resources and biodiversity over a representative portion of a taxon’s historical occurrence. In light of the new genetics information gained since 2010 (Leary 2014, unpublished data), we also believe it is important to acknowledge the moderate to high levels of genetic diversity within the introduced populations in the upper Missouri River basin and the potential adaptive capabilities represented by this diversity. All Arctic grayling populations (introduced or not) currently within the upper Missouri River basin are derived from a common ancestor and have a distinct evolutionary trajectory relative to the historical founding populations in Canada and Alaska. Thus, Arctic grayling originating from and currently within the upper Missouri River basin represent the southernmost assemblage of the species, facing similar selection pressures and evolving independent of more northern populations.
The introduced Arctic grayling populations in the upper Missouri River basin occupy, for the most part, high-elevation habitats that are high-quality because of intact riparian areas and a consistent supply of cool water. Given the predicted effects of climate change in the West (see discussion under “Climate Change” in Factor A below), these types of habitats are the same habitats that the Service would explore for long-term conservation of Arctic grayling, if needed, because they may serve as thermal refugia as temperatures rise and provide greater redundancy in case of catastrophic events.
2. In 2010, the Service concluded there did not appear to be any formally recognized conservation value for the introduced populations of Arctic grayling in the upper Missouri River basin because they were not being used in conservation or restoration programs. This conclusion was based on an interpretation of a National Marine Fisheries Service final policy on the consideration of hatchery-origin fish in Endangered Species Act listing determinations for Pacific salmon and steelhead (anadromous Oncorhynchus spp.) (NMFS 2005, entire).
Until recently, the genetic structure and source of these introduced populations were unknown. Populations with a high likelihood of being Montana origin were used for conservation purposes (e.g., reintroductions) as a precautionary approach to Arctic grayling conservation. Now that the amount of genetic diversity within and among the introduced Arctic grayling populations and their source(s) are known, it is probable these introduced populations could be used in future conservation actions as source stock, if needed.
3. In 2010, the Service indicated there were concerns that introduced, lake-dwelling Arctic grayling populations could pose genetic risks to the native fluvial population (i.e., Big Hole Population) as cited in the Montana Fluvial Arctic Grayling Restoration Plan (“Restoration Plan,” 1995, p. 15). In the Restoration Plan, Arctic grayling populations in Agnes, Schwinegar, Odell, Miner and Mussigbrod lakes were identified as potential threats to the genetic integrity of the Big Hole River population because of hydrologic connectivity between these lakes and the Big Hole River and the potential for genetic mixing.
Recently, genetic analyses have confirmed reproductive isolation among extant Arctic grayling populations in the upper Missouri River basin and within the Big Hole River watershed (Peterson and Ardren 2009, p. 1770; Leary 2014, unpublished data). In addition, multiple historical stockings have occurred in the Big Hole River from other sources within the upper Missouri River basin. Recent genetic analysis found no evidence of a significant genetic contribution from historical stocking on the current genetic structure of Arctic grayling in the Big Hole River (Peterson and Ardren 2009, p. 1768). Thus, we now conclude that the concern that lake-dwelling populations within the Big Hole River watershed could pose genetic risks to the Big Hole River fluvial population appears unfounded.
4. In 2010, the Service concluded that introduced populations of Arctic grayling in the upper Missouri River basin had no conservation value because these populations apparently had been isolated from their original source stock for decades without any supplementation from the wild and were established without any formal genetic consideration to selecting and mating broodstock.
It is now apparent from our review of historical stocking records that many of these introduced populations received multiple stockings from the same source or multiple stockings from several different sources over a wide range of years (MFISH 2014a, unpublished data). Additionally, most individual stockings involved a large number of eggs or fry (up to 1 million for some stockings). Cumulatively, this information suggests several points. First, stockings that used a large number of eggs or fry necessitate that gametes from multiple brood fish were used per stocking, given the physical constraints of number of eggs per unit body size of female Arctic grayling. Second, stockings in most of the introduced populations occurred over many years (up to 60 years in some cases). This indicates different cohorts of Arctic grayling had to be used, since the generation time of Arctic grayling is approximately 3.5 years in the upper Missouri River basin (references in Dehaan et al. 2014, p. 10). Lastly, the new genetic analyses from seven of the introduced Arctic grayling populations indicate moderate to high levels of genetic diversity within the populations. This result could likely only be obtained from the founding of these populations using large numbers of brood fish and gametes over multiple years. Mutation is unlikely to have accounted for these levels of genetic diversity over a relatively short time period of isolation (Freeman and Herron 2001, p. 143).
For perspective, Montana Fish, Wildlife, and Parks has developed guidelines for the establishment and maintenance of Arctic grayling broodstock. To adequately capture most of the genetic variation in a source population, the crossing of a minimum of 25 male and 25 female Arctic grayling is currently recommended (Leary 1991, p. 2151). It is likely that the historical stockings used to found the introduced Arctic grayling populations in the upper Missouri River basin equaled or exceeded this through stocking large numbers of eggs or fry over multiple years.
5. In 2010, the Service concluded that the source populations used to found the introduced Arctic grayling populations in the upper Missouri River drainage were not well documented (Peterson and Ardren 2009, p. 1767), so we could not be certain of whether these Arctic grayling were of local origin.
Since 2010, new genetic information (Leary 2014, unpublished data) and review of historical stocking records (MFISH 2014a, unpublished data) indicate the founding populations used for stocking are local and believed representative of the Upper Missouri River DPS of Arctic grayling, and contain moderate to high levels of genetic diversity.
6. In 2010, the Service concluded the primary intent of culturing and introducing Arctic grayling populations within the upper Missouri River basin was to provide recreational fishing opportunities in high mountain lakes, and that, therefore, these introduced populations had no conservation value.
Since 2010, review of the historical literature indicates adfluvial Arctic grayling populations were presumably stocked both for recreational fishing and conservation purposes (Brown 1943, pp. 26-27; Nelson 1954, p. 341; Vincent 1962, p. 151). Following the drought in the 1930s, conservation stockings of Arctic grayling were advocated because most rivers and streams were dewatered, prompting fish managers to introduce Arctic grayling into habitats with a more consistent supply of cool water (e.g., high-elevation mountain lakes; Brown 1943, pp. 26-27; Nelson 1954, p. 341; Vincent 1962, p. 151).
In conclusion, introduced populations of Arctic grayling established within the upper Missouri River basin, whether they were originally established for recreational fishing or conservation purposes, captured moderate to high levels of genetic diversity of upper Missouri River basin Arctic grayling. The potential adaptive capabilities represented by this genetic diversity have conservation value, particularly in a changing climate. These populations reside in high-quality habitat, the same habitat the Service would look to for long-term conservation, if needed. Thus, the introduced populations of Arctic grayling within the upper Missouri River basin have conservation value, and, therefore, we include them in our analysis of a potential DPS of Arctic grayling.
Origins, Biogeography, and Genetics of Arctic Grayling in North America
North American Arctic grayling are most likely descended from Eurasian Thymallus that crossed the Bering land bridge during or before the Pleistocene glacial period (Stamford and Taylor 2004, pp. 1533, 1546). There were multiple opportunities for freshwater faunal exchange between North America and Asia during the Pleistocene, but genetic divergence between North American and Eurasian Arctic grayling suggests that the species could have colonized North America as early as the mid-late Pliocene (more than 3 million years ago) (Stamford and Taylor 2004, p. 1546). Genetic studies of Arctic grayling using mitochondrial DNA (mtDNA, maternally inherited DNA located in cellular organelles called mitochondria) and microsatellite DNA (repeating sequences of nuclear DNA) have shown that North American Arctic grayling consist of at least three major lineages that originated in distinct Pleistocene glacial refugia (Stamford and Taylor 2004, p. 1533). These three groups include a South Beringia lineage found in western Alaska to northern British Columbia, Canada; a North Beringia lineage found on the North Slope of Alaska, the lower Mackenzie River, and to eastern Saskatchewan; and a Nahanni lineage found in the lower Liard River and the upper Mackenzie River drainage in northeastern British Columbia and southeastern Yukon (Stamford and Taylor 2004, pp. 1533, 1540). Arctic grayling from the upper Missouri River basin were tentatively placed in the North Beringia lineage because a small sample (three individuals) of Montana Arctic grayling shared a mtDNA haplotype (form of the mtDNA) with populations in Saskatchewan and the lower Peace River, British Columbia (Stamford and Taylor 2004, p. 1538).
The existing mtDNA data suggest that Missouri River Arctic grayling share a common ancestry with the North Beringia lineage, but other genetic markers (e.g., allozymes, microsatellites) and biogeographic history indicate that Missouri River Arctic grayling have been physically and reproductively isolated from northern populations for millennia. Pre-glacial colonization of the Missouri River basin by Arctic grayling was possible because the river flowed to the north and drained into the Arctic-Hudson Bay prior to the last glacial cycle (Cross et al. 1986, pp. 374-375; Pielou 1991, pp. 194-195). Low mtDNA diversity observed in a small number of Montana Arctic grayling samples and a shared ancestry with Arctic grayling from the North Beringia lineage suggest a more recent, post-glacial colonization of the upper Missouri River basin. In contrast, microsatellite DNA show substantial divergence between Montana and Saskatchewan (i.e., same putative mtDNA lineage) (Peterson and Ardren 2009, entire). Differences in the frequency and size distribution of microsatellite alleles between Montana populations and two Saskatchewan populations indicate that Montana Arctic grayling have been isolated long enough for mutations (i.e., evolution) to be responsible for the observed genetic differences.
Additional comparison of 21 Arctic grayling populations from Alaska, Canada, and the Missouri River basin using 9 of the same microsatellite loci as Peterson and Ardren (2009, entire) further supports the distinction of Missouri River Arctic grayling relative to populations elsewhere in North America (USFWS, unpublished data). Analyses of these data using two different methods clearly separates sample fish from 21 populations into two clusters: One cluster representing populations from the upper Missouri River basin, and another cluster representing populations from Canada and Alaska (USFWS, unpublished data). These new data, although not yet peer reviewed, support the interpretation that the previous analyses of Stamford and Taylor (2004, entire) underestimated the distinctiveness of Missouri River Arctic grayling relative to other sample populations, likely because of the combined effect of small sample sizes and the lack of variation observed in the Missouri River for the markers used in that study (Stamford and Taylor 2004, pp. 1537-1538). Thus, these recent microsatellite DNA data suggest that Arctic grayling may have colonized the Missouri River before the onset of Wisconsin glaciation (more than 80,000 years ago).
Genetic relationships among native and introduced populations of Arctic grayling in Montana have recently been investigated (Peterson and Ardren 2009, entire). Introduced, lake-dwelling populations of Arctic grayling trace some of their original ancestry to the Centennial Valley (Peterson and Ardren 2009, p. 1767), and stocking of hatchery Arctic grayling did not have a large effect on the genetic composition of the extant native populations (Peterson and Ardren 2009, p. 1768). Differences between native populations of the two Arctic grayling ecotypes (adfluvial, fluvial) are not as large as differences resulting from geography (i.e., drainage of origin). For example, native adfluvial Arctic grayling populations from different lakes are genetically different (Peterson and Ardren 2009, p. 1766).
Habitat
Arctic grayling generally require clear, cold water, and are characterized as belonging to a “coldwater” group of salmonids, which also includes bull trout (Salvelinus confluentus) and Arctic char (Salvelinus alpinus) (Selong et al. 2001, p. 1032). Arctic grayling optimal thermal habitat is between 7 to 17 °C (45 to 63 °F), but becomes unsuitable above 20 °C (68 °F) (Hubert et al. 1985, p. 24). Arctic grayling fry may be more tolerant of high water temperature than adults (LaPerriere and Carlson 1973, p. 30; Feldmeth and Eriksen 1978, p. 2041).
Having a broad, nearly circumpolar distribution, Arctic grayling occupy a variety of habitats including small streams, large rivers, lakes, and even bogs (Northcote 1995, pp. 152-153; Scott and Crossman 1998, p. 303). They may even enter brackish water (less than or equal to 4 parts per thousand salt content) when migrating between adjacent river systems (West et al. 1992, pp. 713-714). Native populations are found at elevations ranging from near sea level, such as in Bristol Bay, Alaska, to high-elevation montane valleys (more than 1,830 meters (m) or 6,000 feet (ft)), such as the Big Hole River and Centennial Valley in southwestern Montana. Despite this broad distribution, Arctic grayling have specific habitat requirements that can constrain their local distributions, especially water temperature and channel gradient. At the local scale, Arctic grayling prefer cold water and are often associated with spring-fed habitats in regions with warmer climates (Vincent 1962, p. 33). Arctic grayling are generally not found in swift, high-gradient streams, and Vincent (1962, pp. 36-37, 41-43) characterized typical Arctic grayling habitat in Montana (and Michigan) as low-to-moderate gradient (less than 4 percent) streams and rivers with low-to-moderate water velocities (less than 2 feet/sec (60 centimeters/sec)). Juvenile and adult Arctic grayling in streams and rivers spend much of their time in pool habitat (Kaya 1990 and references therein, p. 20; Lamothe and Magee 2003, pp. 13-14).
Breeding
Arctic grayling typically spawn in the spring or early summer, depending on latitude and elevation (Northcote 1995, p. 149). In Montana, Arctic grayling generally spawn from late April to mid-May by depositing adhesive eggs over gravel substrate without excavating a nest (Kaya 1990, p. 13; Northcote 1995, p. 151). In general, the reproductive ecology of Arctic grayling differs from other salmonid species (trout and salmon) in that Arctic grayling eggs tend to be comparatively small; thus, they have higher relative fecundity (females have more eggs per unit body size). Males establish and defend spawning territories rather than defending access to females (Northcote 1995, pp. 146, 150-151). The time required for development of eggs from embryo until they emerge from stream gravel and become swim-up fry depends on water temperature (Northcote 1995, p. 151). In the upper Missouri River basin, development from embryo to fry averages about 3 weeks (Kaya 1990, pp. 16-17). Small, weakly swimming fry (typically 1-1.5 centimeters (cm) (0.4-0.6 in.) at emergence) prefer low-velocity stream habitats (Armstrong 1986, p. 6; Kaya 1990, pp. 23-24; Northcote 1995, p. 151).
Arctic grayling of all ages feed primarily on aquatic and terrestrial invertebrates captured on or near the water surface, but also will feed opportunistically on fish and fish eggs (Northcote 1995, pp. 153-154; Behnke 2002, p. 328). Feeding locations for individual fish are typically established and maintained through size-mediated dominance hierarchies where larger individuals defend favorable feeding positions (Hughes 1992, p. 1996).
General Life History Diversity
Migratory behavior is a common life-history trait in salmonid fishes such as Arctic grayling (Armstrong 1986, pp. 7-8; Northcote 1995, pp. 156-158; 1997, pp. 1029, 1031-1032, 1034). In general, migratory behavior in Arctic grayling and other salmonids results in cyclic patterns of movement between refuge, rearing-feeding, and spawning habitats (Northcote 1997, p. 1029).
Arctic grayling may move to refuge habitat as part of a regular seasonal migration (e.g., in winter), or in response to episodic environmental stressors (e.g., high summer water temperatures). In Alaska, Arctic grayling in rivers typically migrate downstream in the fall, moving into larger streams or mainstem rivers that do not completely freeze (Armstrong 1986, p. 7). In Arctic rivers, fish often seek overwintering habitat influenced by groundwater (Armstrong 1986, p. 7). In some drainages, individual fish may migrate considerable distances (greater than 150 km or 90 mi) to overwintering habitats (Armstrong 1986, p. 7). In the Big Hole River, Montana, similar downstream and long-distance movement to overwintering habitat has been observed in Arctic grayling (Shepard and Oswald 1989, pp. 18-21, 27). In addition, Arctic grayling in the Big Hole River may move downstream in proximity to colder tributary streams in summer when thermal conditions in the mainstem river become stressful (Lamothe and Magee 2003, p. 17).
In spring, mature Arctic grayling leave overwintering areas and migrate to suitable spawning sites. In river systems, this typically involves an upstream migration to tributary streams or shallow riffles within the mainstem (Armstrong 1986, p. 8; Shepard and Oswald 1989; p. 18). Arctic grayling in lakes typically migrate to either the inlet or outlet to spawn (Armstrong 1986, p. 8; Kaya 1989, p. 474; Northcote 1995 p. 148). In some situations, Arctic grayling exhibit natal homing, whereby individuals spawn in or near the location where they were born (Northcote 1995 pp. 157-160; Boltz and Kaeding 2002, p. 22); however, it is unclear what factors may be influencing the extent of this phenomenon.
Fry from river populations typically seek feeding and rearing habitats in the vicinity of where they were spawned (Armstrong 1986, pp. 6-7; Kaya and Jeanes 1995, p. 455; Northcote 1995, p. 156), while those from lake populations migrate downstream (inlet spawners) or upstream (outlet spawners) to the adjacent lake. Following spawning, adults move to appropriate feeding areas if they are not adjacent to spawning habitat (Armstrong 1986, pp. 7-8; Shepard and Oswald 1989; p. 18). Juvenile Arctic grayling may undertake seasonal migrations between feeding and overwintering habitats until they reach maturity and add the spawning migration to this cycle (Northcote 1995, pp. 156-157).
Life History Diversity in Arctic Grayling in the Upper Missouri River Basin
Two general life-history forms or ecotypes of native Arctic grayling occur in the upper Missouri River Arctic: Fluvial and adfluvial. Fluvial fish use river or stream (lotic) habitat for all of their life cycles and may undergo extensive migrations within river habitat, up to 50 miles in the Big Hole River in Montana (Shepard and Oswald 1989, p. 18). Adfluvial fish live in lakes and migrate to tributary streams to spawn. These same life-history forms also are expressed by Arctic grayling elsewhere in North America (Northcote 1997, p. 1030). Historically, the fluvial life-history form predominated in the Missouri River basin above the Great Falls, perhaps because there were only a few lakes accessible to natural colonization of Arctic grayling that would permit expression of the adfluvial ecotype (Kaya 1992, p. 47). The fluvial and adfluvial life-history forms of Arctic grayling in the upper Missouri River do not appear to represent distinct evolutionary lineages. Instead, they appear to represent an example of adaptive radiation (Schluter 2000, p. 1), whereby the forms differentiated from a common ancestor and developed traits that allowed them to exploit different habitats. The primary evidence for this conclusion is genetic data that indicate that within the Missouri River basin the two ecotypes are more closely related to each other than they are to the same ecotype elsewhere in North America (Redenbach and Taylor 1999, pp. 27-28; Stamford and Taylor 2004, p. 1538; Peterson and Ardren 2009, p. 1766). Historically, there may have been some genetic exchange between the two life-history forms as individuals strayed or dispersed into different populations (Peterson and Ardren 2009, p. 1770), but the genetic structure of current populations in the upper Missouri River basin is consistent with reproductive isolation.
The fluvial and adfluvial forms of Arctic grayling appear to differ in their genetic characteristics, but there appears to be some plasticity in behavior where individuals from a population can exhibit a range of behaviors. Arctic grayling fry in Montana can exhibit heritable, genetically-based differences in swimming behavior between fluvial and adfluvial ecotypes (Kaya 1991, pp. 53, 56-58; Kaya and Jeanes 1995, pp. 454, 456). Progeny of Arctic grayling from the fluvial ecotype exhibited a greater tendency to hold their position in flowing water relative to progeny from adfluvial ecotypes (Kaya 1991, pp. 53, 56-58; Kaya and Jeanes 1995, pp. 454, 456). Similarly, young Arctic grayling from inlet and outlet spawning adfluvial ecotypes exhibited an innate tendency to move downstream and upstream, respectively (Kaya 1989, pp. 478-480). All three studies (Kaya 1989, entire; 1991, entire; Kaya and Jeanes 1995, entire) demonstrate that the response of fry to flowing water depended strongly on the life-history form (ecotype) of the source population, and that this behavior has a genetic basis. However, behavioral responses also were mediated by environmental conditions (light—Kaya 1991, pp. 56-57; light and water temperature—Kaya 1989, pp. 477-479), and some progeny of each ecotype exhibited behavior characteristic of the other; for example some individuals from the fluvial ecotype moved downstream rather than holding position, and some individuals from an inlet-spawning adfluvial ecotype held position or moved upstream (Kaya 1991, p. 58). These observations indicate that some plasticity for behavior exists, at least for very young Arctic grayling.
The ability of the fluvial ecotype to give rise to a functional population of the adfluvial ecotype has been demonstrated. Most extant adfluvial Arctic grayling populations in the Upper Missouri River originated from fluvial-dominated sources (see Table 1; Kaya 1992, p. 53; Jeanes 1996, pp. 54). However, the ability of the adfluvial ecotype to give rise to a functional population of fluvial ecotype is less certain. Circumstantial support for reduced plasticity in adfluvial Arctic grayling comes from observations that adfluvial fish stocked in river habitats almost never establish populations (Kaya 1990, pp. 31-34). However, we note that adfluvial Arctic grayling retain some life-history flexibility—at least in lake environments—as naturalized populations derived from inlet-spawning stocks have established outlet-spawning demes (a deme is a local populations that shares a distinct gene pool) in Montana and in Yellowstone National Park (Kruse 1959, p. 318; Kaya 1989, p. 480). In addition, a small percentage of young adfluvial Arctic grayling exposed to flow exhibited fluvial-like characteristics (e.g., station-holding or upstream movement) in a laboratory experiment designed to assess movement tendencies of adfluvial and fluvial Arctic grayling in flowing water (Kaya 1991, p. 56). These results indicate some plasticity exists in adfluvial Arctic grayling that may allow some progeny of adfluvial individuals to express a fluvial life history. Nonetheless, the frequent failure of introductions of adfluvial Arctic grayling into fluvial habitats suggest a cautionary approach to the loss of particular life-history forms is warranted.
Age and Growth
Age at maturity and longevity in Arctic grayling varies regionally and is probably related to growth rate, with populations in colder, northern latitudes maturing at later ages and having a greater lifespan (Kruse 1959, pp. 340-341; Northcote 1995 and references therein, pp. 155-157). Arctic grayling in the upper Missouri River typically mature at age 2 (males) or age 3 (females), and individuals greater than age 6 are rare (Kaya 1990, p. 18; Magee and Lamothe 2003, pp. 16-17). The majority of the Arctic grayling spawning in two tributaries in the Centennial Valley, Montana, were age 3, and the oldest individuals aged from a larger sample were age 6 (Nelson 1954, pp. 333-334). Arctic grayling spawning in Red Rock Creek were mostly ages 2 to 5, but some individuals were age 7 (Mogen 1996, pp. 32-34).
Generally, growth rates of Arctic grayling are greatest during the first years of life then slow dramatically after maturity. Within that general pattern, there is substantial variation among populations from different regions. Arctic grayling populations in Montana (Big Hole River and Red Rock Lakes) have very high growth rates relative to those from British Columbia, Asia, and the interior and North Slope of Alaska (Carl et al. 1992, p. 240; Northcote 1995, pp. 155-157; Neyme 2005, p. 28).
Distinct Vertebrate Population Segment
Under the Service’s Policy Regarding the Recognition of Distinct Vertebrate Population Segments Under the Endangered Species Act (61 FR 4722; February 7, 1996), three elements are considered in the decision concerning the establishment and classification of a possible DPS. These are applied similarly for additions to or removal from the Federal List of Endangered and Threatened Wildlife. These elements include:
(1) The discreteness of a population in relation to the remainder of the species to which it belongs;
(2) The significance of the population segment to the species to which it belongs; and
(3) The population segment’s conservation status in relation to the Act’s standards for listing, delisting, or reclassification (i.e., is the population segment endangered or threatened).
Discreteness
Under the DPS policy, a population segment of a vertebrate taxon may be considered discrete if it satisfies either one of the following conditions:
(1) It is markedly separated from other populations of the same taxon as a consequence of physical, physiological, ecological, or behavioral factors. Quantitative measures of genetic or morphological discontinuity may provide evidence of this separation.
(2) It is delimited by international governmental boundaries within which differences in control of exploitation, management of habitat, conservation status, or regulatory mechanisms exist that are significant in light of section 4(a)(1)(D) of the Act.
Arctic grayling native to the upper Missouri River are isolated from all other populations of the species, which inhabit the Arctic Ocean, Hudson Bay, and north Pacific Ocean drainages in Asia and North America. Arctic grayling native to the upper Missouri River occur as a disjunct group of populations approximately 800 km (500 mi) to the south of the next-nearest Arctic grayling population in central Alberta, Canada. Missouri River Arctic grayling have been isolated from other populations for at least 10,000 years based on historical reconstruction of river flows at or near the end of the Pleistocene (Cross et al. 1986, p. 375; Pileou 1991, pp. 10-11). Genetic data confirm Arctic grayling in the Missouri River basin have been reproductively isolated from populations to the north for millennia (Everett 1986, pp. 79-80; Redenbach and Taylor 1999, p. 23; Stamford and Taylor 2004, p. 1538; Peterson and Ardren 2009, pp. 1764-1766; USFWS, unpublished data). Consequently, we conclude that Arctic grayling native to the upper Missouri River are markedly separated from other native populations of the taxon as a result of physical factors (isolation), and therefore meet the first criterion of discreteness under the DPS policy. As a result, Arctic grayling native to the upper Missouri River are considered a discrete population according to the DPS policy. Because the entity meets the first criterion (markedly separated), an evaluation with respect to the second criterion (international boundaries) is not needed.
Significance
If a population segment is considered discrete under one or more of the conditions described in the Service’s DPS policy, its biological and ecological significance will be considered in light of Congressional guidance that the authority to list DPSs be used “sparingly” while encouraging the conservation of genetic diversity. In making this determination, we consider available scientific evidence of the discrete population segment’s importance to the taxon to which it belongs. Since precise circumstances are likely to vary considerably from case to case, the DPS policy does not describe all the classes of information that might be used in determining the biological and ecological importance of a discrete population. However, the DPS policy describes four possible classes of information that provide evidence of a population segment’s biological and ecological importance to the taxon to which it belongs. As specified in the DPS policy (61 FR 4722), this consideration of the population segment’s significance may include, but is not limited to, the following:
(1) Persistence of the discrete population segment in an ecological setting unusual or unique to the taxon;
(2) Evidence that loss of the discrete population segment would result in a significant gap in the range of a taxon;
(3) Evidence that the discrete population segment represents the only surviving natural occurrence of a taxon that may be more abundant elsewhere as an introduced population outside its historical range; or
(4) Evidence that the discrete population segment differs markedly from other populations of the species in its genetic characteristics.
A population segment needs to satisfy only one of these conditions to be considered significant. Furthermore, other information may be used as appropriate to provide evidence for significance.
Unique Ecological Setting
Water temperature is a key factor influencing the ecology and physiology of ectothermic (body temperature regulated by ambient environmental conditions) salmonid fishes, and can dictate reproductive timing, growth and development, and life-history strategies. Groundwater temperatures can be related to air temperatures (Meisner 1990, p. 282), and thus reflect the regional climatic conditions. Warmer groundwater influences ecological factors such as food availability, the efficiency with which food is converted into energy for growth and reproduction, and ultimately growth rates of aquatic organisms (Allan 1995, pp. 73-79). Aquifer structure and groundwater temperature is important to salmonid fishes because groundwater can strongly influence stream temperature, and consequently egg incubation and fry growth rates, which are strongly temperature-dependent (Coutant 1999, pp. 32-52; Quinn 2005, pp. 143-150).
Missouri River Arctic grayling occur within the 4 to 7 °C (39 to 45 °F) ground water isotherm (see Heath 1983, p. 71; an isotherm is a line connecting bands of similar temperatures on the earth’s surface), whereas most other North American Arctic grayling are found in isotherms less than 4 °C, and much of the species’ range is found in areas with discontinuous or continuous permafrost (Meisner et al. 1988, p. 5; Table 2). Much of the historical range of Arctic grayling in the upper Missouri River is encompassed by mean annual air temperature isotherms of 5 to 10 °C (41 to 50 °F) (USGS 2009), with the colder areas being in the headwaters of the Madison River in Yellowstone National Park. In contrast, Arctic grayling in Canada, Alaska, and Asia are located in regions encompassed by air temperature isotherms 5 °C and colder (41 °F and colder), with much of the species distributed within the 0 to −10 °C isolines (32 to 14 °F). This difference is significant because Arctic grayling in the Missouri River basin have evolved in isolation for millennia in a generally warmer climate than other populations. The potential for thermal adaptations makes Missouri River Arctic grayling a significant biological resource for the species under expected climate change scenarios.
Ecological setting variable | Missouri River | Rest of taxon |
---|---|---|
Bailey’s Ecoregion | Dry Domain: Temperate Steppe | Polar Domain: Tundra & Subarctic Humid Temperate: Marine, Prairie, Warm Continental Mountains. |
Air temperature (isotherm) | 5 to 10 °C (41 to 50 °F) | −15 to 5 °C (5 to 41 °F). |
Groundwater temperature (isotherm) | 4 to 7 °C (39 to 45 °F) | Less than 4 °C (Less than 39 °F). |
Arctic grayling in the upper Missouri River basin occur in a temperate ecoregion distinct from all other Arctic grayling populations worldwide, which occur in Arctic or sub-Arctic ecoregions dominated by Arctic flora and fauna. An ecoregion is a continuous geographic area within which there are associations of interacting biotic and abiotic features (Bailey 2005, pp. S14, S23). These ecoregions delimit large areas within which local ecosystems recur more or less in a predictable fashion on similar sites (Bailey 2005, p. S14). Ecoregional classification is hierarchical, and based on the study of spatial coincidences, patterning, and relationships of climate, vegetation, soil, and landform (Bailey 2005, p. S23). The largest ecoregion categories are domains, which represent subcontinental areas of similar climate (e.g., polar, humid temperate, dry, and humid tropical) (Bailey 1994; 2005, p. S17). Domains are divided into divisions that contain areas of similar vegetation and regional climates. Arctic grayling in the upper Missouri River basin are the only example of the species naturally occurring in a dry domain (temperate steppe division; Table 2). The vast majority of the species’ range is found in the polar domain (all of Asia, most of North America), with small portions of the range occurring in the humid temperate domain (northern British Columbia and southeast Alaska). Occupancy of Missouri River Arctic grayling in a temperate ecoregion is significant for two primary reasons. First, an ecoregion represents a suite of factors (climate, vegetation, landform) influencing, or potentially influencing, the evolution of species within that ecoregion. Since Missouri River Arctic grayling have existed for thousands of years in an ecoregion quite different from the majority of the taxon, they have likely developed adaptations during these evolutionary timescales that distinguish them from the rest of the taxon, even if we have yet to conduct the proper studies to measure these adaptations. Second, the occurrence of Missouri River Arctic grayling in a unique ecoregion helps reduce the risk of species-level extinction, as the different regions may respond differently to environmental change.
Arctic grayling in the upper Missouri River basin have existed for at least 10,000 years in an ecological setting quite different from that experienced by Arctic grayling elsewhere in the species’ range. The most salient aspects of this different setting relate to temperature and climate, which can strongly and directly influence the biology of ectothermic species (like Arctic grayling). Arctic grayling in the upper Missouri River have experienced warmer temperatures than most other populations. Physiological and life-history adaptation to local temperature regimes are regularly documented in salmonid fishes (Taylor 1991, pp. 191-193), but experimental evidence for adaptations to temperature, such as unusually high temperature tolerance or lower tolerance to colder temperatures, is lacking for Missouri River Arctic grayling because the appropriate studies have not been conducted. Lohr et al. (1996, p. 934) studied the upper thermal tolerances of Arctic grayling from the Big Hole River, but their research design did not include other populations from different thermal regimes, so it was not possible to make between-population contrasts under a common set of conditions. Arctic grayling from the upper Missouri River demonstrate very high growth rates relative to other populations (Northcote 1995, p. 157). Experimental evidence obtained by growing fish from populations under similar conditions would be needed to measure the relative influence of genetics (local adaptation) versus environment.
We conclude that the occurrence of Arctic grayling in the upper Missouri River is biogeographically important to the species, that grayling there have occupied a warmer and more temperate setting that is distinctly different from the ecological settings relative to the rest of the species (see Table 2, above), and that they have been on a different evolutionary trajectory for at least 10,000 years. We conclude that these differences are significant because they may provide the species with additional evolutionary resiliency in the future in light of the changing climate. Consequently, we believe that Arctic grayling in the upper Missouri River occupy a unique ecological setting for the species.
Gap in the Range
Arctic grayling in Montana (southern extent is approximately 44°36′23″ N latitude) represent the southern-most extant population of the species’ distribution since the Pleistocene glaciation. The next-closest native Arctic grayling population outside the Missouri River basin is found in the Pembina River (approximately 52°55′6.77″ N latitude) in central Alberta, Canada, west of Edmonton (Blackburn and Johnson 2004, pp. ii, 17; ASRD 2005, p. 6). The Pembina River drains into Hudson Bay and is thus disconnected from the Missouri River basin. Loss of the native Arctic grayling of the upper Missouri River would shift the southern distribution of Arctic grayling by more than 8° latitude (about 500 miles). Such a dramatic range constriction would constitute a significant geographic gap in the species’ range and would eliminate a genetically distinct group of Arctic grayling, which may limit the species’ ability to cope with future environmental change.
Marginal populations, defined as those on the periphery of the species’ range, are believed to have high conservation significance (Mitikka et al. 2008; Gibson et al. 2009, entire; Haak et al. 2010, entire; Osborne et al. 2012). Peripheral populations may occur in suboptimal habitats and thus be subjected to very strong selective pressures (Fraser 2000, p. 50). Consequently, individuals from these populations may contain adaptations that may be important to the taxon in the future. Lomolino and Channell (1998, p. 482) hypothesize that because peripheral populations should be adapted to a greater variety of environmental conditions, then they may be better suited to deal with anthropogenic (human-caused) disturbances than populations in the central part of a species’ range. Arctic grayling in the upper Missouri River have, for millennia, existed in a climate warmer than that experienced by the rest of the taxon. If this selective pressure has resulted in adaptations to cope with increased water temperatures, then the population segment may contain genetic resources important to the taxon. For example, if northern populations of Arctic grayling are less suited to cope with increased water temperatures expected under climate warming, then Missouri River Arctic grayling might represent an important population for reintroduction in those northern regions. We believe that Arctic grayling’s occurrence at the southernmost extreme of the range in the upper Missouri River contributes to the resilience of the overall taxon because these peripheral populations may possess increased adaptability relative to the rest of the taxon.
Only Surviving Natural Occurrence of the Taxon That May Be More Abundant Elsewhere as an Introduced Population Outside of Its Historical Range
This criterion does not directly apply to the Arctic grayling in the upper Missouri River because it is not the only surviving natural occurrence of the taxon; there are native Arctic grayling populations in Canada, Alaska, and Asia.
Differs Markedly in Its Genetic Characteristics
Differences in genetic characteristics can be measured at the molecular, genetic, or phenotypic level. Three different types of molecular markers (allozymes, mtDNA, and microsatellites) demonstrate that Arctic grayling from the upper Missouri River are genetically different from those in Canada, Alaska, and Asia (Everett 1986, pp. 79-80; Redenbach and Taylor 1999, p. 23; Stamford and Taylor 2004, p. 1538; Peterson and Ardren 2009, pp. 1764-1766; USFWS, unpublished data). These data confirm the reproductive isolation among populations that establishes the discreteness of Missouri River Arctic grayling under the DPS policy. Here, we speak to whether these data also establish significance.
Allozymes
Using allozyme data, Everett (1986, entire) found marked genetic differences among Arctic grayling collected from the Chena River in Alaska; those descended from fish native to the Athabasca River drainage in the Northwest Territories, Canada; and native upper Missouri River drainage populations or populations descended from them (see Leary 2005, pp. 1-2). The Canadian population had a high frequency of two unique alleles (forms of a gene), which strongly differentiated them from all the other samples (Everett 1986, p. 44). With the exception of one introduced population in an irrigation canal (Sunnyslope canal) in Montana that is believed to have experienced extreme genetic bottlenecks, the Chena River (Alaskan) fish were highly divergent from all the other samples as they possessed an unusually low frequency of a specific allele (Everett 1986, p. 60; Leary 2005, p. 1), and contained a unique variant of another allele (Leary 2005, p. 1). Overall, each of the four native Missouri River populations examined (Big Hole, Miner, Mussigbrod, and Centennial Valley) exhibited statistically significant differences in allele frequencies relative to both the Chena River (Alaska) and Athabasca River (Canada) populations (Everett 1986, pp. 15, 67).
Combining the data of Everett (1986, entire), Hop and Gharrett (1989, entire), and Leary (1990, entire) provides information from 21 allozyme loci (genes) from five native upper Missouri River drainage populations, five native populations in the Yukon River drainage in Alaska, and the one population descended from the Athabasca River drainage in Canada (Leary 2005, pp. 1-2). Examination of the genetic variation in these samples indicated that most of the genetic divergence is due to differences among drainages (29 percent) and comparatively little (5 percent) results from differences among populations within a drainage (Leary 2005, p. 1).
Mitochondrial DNA
Analysis using mtDNA indicates that Arctic grayling in North America represent at least three evolutionary lineages that are associated with distinct glacial refugia (Redenbach and Taylor 1999, entire; Stamford and Taylor 2004, entire). Arctic grayling in the upper Missouri River basin belong to the so-called North Beringia lineage (Redenbach and Taylor 1999, pp. 27-28; Samford and Taylor 2004, pp. 1538-1540) because they possess a form of mtDNA that was generally absent from populations collected from other locations within the species’ range in North America (Redenbach and Taylor 1999, pp. 27-28; Stamford and Taylor 2004, p. 1538). The notable exceptions were that some fish from the lower Peace River drainage in British Columbia, Canada, and all sampled individuals from the Saskatchewan River drainage Saskatchewan, Canada, also possessed this form of mtDNA (Stamford and Taylor 2004, p. 1538).
A form of mtDNA common in upper Missouri River Arctic grayling, which occurs at lower frequencies in other populations, indicates that Arctic grayling native to the upper Missouri River drainage probably originated from a glacial refuge in the drainage and subsequently migrated northwards when the Missouri River temporarily flowed into the Saskatchewan River and was linked to an Arctic drainage (Cross et al. 1986, pp. 374-375; Pielou 1991, p. 195). When the Missouri River began to flow southwards because of the advance of the Laurentide ice sheet (Cross et al. 1986, p. 375; Pileou 1991, p. 10), the Arctic grayling in the drainage became physically and reproductively isolated from the rest of the species’ range (Leary 2005, p. 2; Campton 2004, p. 6), which would have included those populations in Saskatchewan. Alternatively, the Missouri River Arctic grayling could have potentially colonized Saskatchewan or the Lower Peace River (in British Columbia) or both post-glacially (Stamford 2001, p. 49) via a gap in the Cordilleran and Laurentide ice sheets (Pielou 1991, pp. 10-11), which also might explain the low frequency ‘Missouri River” mtDNA in Arctic grayling in the Lower Peace River and Upper Yukon River.
We do not interpret the observation that Arctic grayling in Montana and Saskatchewan, and to lesser extent those from the Lower Peace and Upper Yukon River systems, share a mtDNA haplotype to mean that these groups of fish are genetically identical. Rather, we interpret it to mean that these fish shared a common ancestor tens to hundreds of thousands of years ago.
Microsatellite DNA
Recent analysis of microsatellite DNA (highly variable portions of nuclear DNA) showed substantial divergence between Arctic grayling in Missouri River and Saskatchewan populations (Peterson and Ardren 2009, entire). This divergence between populations was measured in terms of allele frequencies, using a metric called F
st (Allendorf and Luikart 2007, pp. 52-54, 198-199). An analogous metric, named R
st, also measures genetic differentiation between populations based on microsatellite DNA, but differs from F
st in that it also considers the size differences between alleles (Hardy et al. 2003, p. 1468). An F
st or R
st of 0 indicates that populations are the same genetically, whereas a value of 1 indicates the populations share no genetic material at the markers being surveyed. F
st values range from 0.13 to 0.31 (average 0.18) between Missouri River and Saskatchewan populations (Peterson and Ardren 2009, pp. 1758, 1764-1765), whereas R
st values range from 0.47 to 0.71 (average 0.54) for the same comparisons (Peterson and Ardren 2009, pp. 1758, 1764-1765). These values indicate that the two populations differ significantly in allele frequency and also in the size of those alleles. This outcome indicates that the observed genetic differences are due to mutational differences, which suggests the groups may have been separated for millennia (Peterson and Ardren 2009, pp. 1767-1768).
Analysis of Arctic grayling populations from Alaska, Canada, and the Missouri River basin using nine of the same microsatellite loci as Peterson and Ardren (2009, entire) further supports the distinction of Missouri River Arctic grayling relative to populations elsewhere in North America (USFWS, unpublished data). This analysis clearly separated sample fish from 21 populations into two clusters: One cluster representing populations from the upper Missouri River basin, and another cluster representing populations from across Canada and Alaska (USFWS, unpublished data). Divergence in size among these alleles further supports the distinction between Missouri River Arctic grayling and those in Canada and Alaska (USFWS, unpublished data). The interpretation of these data is that the Missouri River populations and the Canada/Alaska populations are highly genetically distinct at the microsatellite loci considered.
Phenotypic Characteristics Influenced by Genetics—Meristics
Phenotypic variation can be evaluated by counts of body parts (i.e., meristic counts of the number of gill rakers, fin rays, and vertebrae characteristics of a population) that can vary within and among species. These meristic traits are influenced by both genetics and the environment (Allendorf and Luikart 2007, pp. 258-259). When the traits are controlled primarily by genetic factors, then meristic characteristics can indicate significant genetic differences among groups. Arctic grayling north of the Brooks Range in Alaska and in northern Canada had lower lateral line scale counts than those in southern Alaska and Canada (McCart and Pepper 1971, entire). These two scale-size phenotypes are thought to correspond to fish from the North and South Beringia glacial refuges, respectively (Stamford and Taylor 2004, p. 1545). Arctic grayling from the Centennial Valley had a phenotype intermediate to the large- and small-scale types (McCart and Pepper 1971, pp. 749, 754). Arctic grayling populations from the Missouri River (and one each from Canada and Alaska) could be correctly assigned to their group 60 percent of the time using a suite of seven meristic traits (Everett 1986, pp. 32-35). Those native Missouri River populations that had high genetic similarity also tended to have similar meristic characteristics (Everett 1986, pp. 80, 83).
Arctic grayling from the Big Hole River showed marked differences in meristic characteristics relative to two populations from Siberia, and were correctly assigned to their population of origin 100 percent of the time (Weiss et al. 2006, pp. 512, 515-516, 518). The populations that were significantly different in terms of their meristic characteristics also exhibited differences in molecular genetic markers (Weiss et al. 2006, p. 518).
Inference Concerning Genetic Differences in Arctic Grayling of the Missouri River Relative to Other Examples of the Taxon
We believe the differences between Arctic grayling in the Missouri River and sample populations from Alaska and Canada measured using allozymes (Everett 1986, entire; Leary 2005, entire), mitochondrial DNA (Redenbach and Taylor 1999, entire; Stamford and Taylor 2004, entire), and microsatellite DNA markers (Peterson and Ardren 2009, pp. 1764-1766; USFWS, unpublished data) represent “marked genetic differences” in terms of the extent of differentiation (e.g., F
st, R
st) and the importance of that genetic legacy to the rest of the taxon. The presence of morphological characteristics separating Missouri River Arctic grayling from other populations also likely indicates genetic differences, although this conclusion is based on a limited number of populations (Everett 1986, pp. 32-35; Weiss et al. 2006, entire), and we cannot entirely rule out the influence of environmental variation.
The intent of the DPS policy and the Act is to preserve important elements of biological and genetic diversity, not necessarily to preserve the occurrence of unique alleles in particular populations. In Arctic grayling of the Missouri River, the microsatellite DNA data indicate that the group is evolving independently from the rest of the species. The extirpation of this group would mean the loss of the genetic variation in one of the two most distinct groups identified in the microsatellite DNA analysis, and the loss of the future evolutionary potential that goes with it. Thus, the genetic data support the conclusion that Arctic grayling of the upper Missouri River represent a unique and irreplaceable biological resource of the type the Act was intended to preserve. Thus, we conclude that Missouri River Arctic grayling differ markedly in their genetic characteristics relative to the rest of the taxon.
Upper Missouri River Arctic grayling satisfy the significance criteria outlined in the Services’ DPS policy because they occur in a unique ecological setting, are separated from other Arctic grayling populations by a large gap in their range, and differ markedly in their genetic characteristics relative to other Arctic grayling populations. Therefore, we consider the Arctic grayling in the upper Missouri River basin significant to the taxon to which it belongs under the Service’s DPS policy.
Determination of Distinct Population Segment
We find that a population segment that includes all native ecotypes of Arctic grayling in the upper Missouri River basin satisfies the discreteness standard of the DPS policy. The segment is physically isolated, and genetic data indicate that Arctic grayling in the Missouri River basin have been separated from other populations for thousands of years. The population segment occurs in an isolated geographic area far south of all other Arctic grayling populations worldwide, and we find that loss of this population segment would create a significant gap in the species’ range. Molecular genetic data clearly differentiate Missouri River Arctic grayling from other Arctic grayling populations, including those in Canada and Alaska.
Based on the best scientific and commercial information available, as described above, we find that, under the Service’s DPS policy, upper Missouri River Arctic grayling are discrete and are significant to the taxon to which they belong. Because the upper Missouri River population of Arctic grayling is both discrete and significant, it qualifies as a DPS under the Act.
As we described above, we are including introduced Arctic grayling populations that occur in lakes in the upper Missouri River basin as part of the DPS. The Service has interpreted the Act to provide a statutory directive to conserve species in their native ecosystems (49 FR 33885; August 27, 1984) and to conserve genetic resources and biodiversity over a representative portion of a taxon’s historical occurrence (61 FR 4722; February 7, 1996). The introduced Arctic grayling populations occur within the boundaries of the upper Missouri River basin and represent moderate to high levels of genetic diversity from within the basin. The future adaptive capabilities represented by this genetic diversity have conservation value, particularly given a changing climate.
We define the historical range of this population segment to include the major streams, lakes, and tributary streams of the upper Missouri River (mainstem Missouri, Smith, Sun, Beaverhead, Jefferson, Big Hole, and Madison Rivers, as well as their key tributaries, as well as a few small lakes where Arctic grayling are or were believed to be native (Elk Lake, Red Rock Lakes in the Centennial Valley, Miner Lake, and Mussigbrod Lake, all in Beaverhead County, Montana)). We define the current range of the DPS to consist of extant native populations in the Big Hole River, Miner Lake, Mussigbrod Lake, Madison River-Ennis Reservoir, and Centennial Valley, as well as all known introduced populations within the upper Missouri River basin. We refer to this entity as the Upper Missouri River DPS of Arctic grayling. The remainder of this finding will thus focus on the population status of and potential threats to this entity.
Population Status and Trends of Populations in the Upper Missouri River DPS
The Upper Missouri River DPS of Arctic grayling is comprised of 20 populations, including 2 fluvial populations and 16 adfluvial populations. Two other populations (Centennial Valley and Madison River/Ennis Reservoir) appear to exhibit both fluvial and adfluvial components (Table 3). Arctic grayling from the Centennial Valley (Long Creek) and Ennis Reservoir/Madison River (mainstem Madison River) have been documented well past the spawning period through autumn. These occurrences are more prevalent in Long Creek in the Centennial Valley than in the Madison River population and do not appear to be linked to individual Arctic grayling seeking thermal refugia during summer (Montana Arctic Grayling Workgroup (AGW) 1995; p. 1; Cayer 2014a, pers. comm.; MFISH 2014b, unpublished data). These occurrences include multiple age classes (Age-1 to Age-3) of Arctic grayling in both Long Creek and the Madison River and are located in stream reaches that are considerable distances (up to 15 miles in the Madison River) from adfluvial habitats (Cayer 2014a, pers. comm.; MFISH 2014b, unpublished data). Eighteen of the 20 populations occur solely on Federal or majority Federal land; the remaining two (Big Hole River and Ennis Reservoir/Madison River) occur on primarily private land.
BILLING CODE 4310-55-P
BILLING CODE 4310-55-C
Estimated abundance of reproductively mature individuals in the two fluvial populations varies from about one hundred to several thousand Arctic grayling (Table 3). Where quantitative data are available, estimated abundance of mature individuals in adfluvial populations (including the two populations exhibiting both life histories) varies from a few hundred to around 25,000 Arctic grayling. Most populations are currently stable or increasing in abundance, with the exception of the Ennis Reservoir/Madison River population (Table 3).
Distinct Population Segment Five-Factor Analysis
Since the Arctic grayling in the upper Missouri River basin qualifies as a DPS, we will now evaluate its status with regard to its potential for listing as endangered or threatened based on the five factors enumerated in section 4(a) of the Act. Our evaluation of the Upper Missouri River DPS of Arctic grayling follows.
Summary of Information Pertaining to the Five Factors Back to Top
Section 4 of the Act (16 U.S.C. 1533) and implementing regulations (50 CFR 424) set forth procedures for adding species to, removing species from, or reclassifying species on the Federal Lists of Endangered and Threatened Wildlife and Plants. Under section 4(a)(1) of the Act, a species may be determined to be endangered or threatened based on any of the following five factors:
(A) The present or threatened destruction, modification, or curtailment of its habitat or range;
(B) Overutilization for commercial, recreational, scientific, or educational purposes;
(C) Disease or predation;
(D) The inadequacy of existing regulatory mechanisms; or
(E) Other natural or manmade factors affecting its continued existence.
In making this finding, information pertaining to the Upper Missouri River DPS of Arctic grayling in relation to the five factors provided in section 4(a)(1) of the Act is discussed below. In considering what factors might constitute threats, we must look beyond the mere exposure of the species to the factor to determine whether the species responds to the factor in a way that causes actual impacts to the species. If there is exposure to a factor, but no response, or only a positive response, that factor is not a threat. If there is exposure and the species responds negatively, the factor may be a threat and we then attempt to determine how significant a threat it is. If the threat is significant, it may drive or contribute to the risk of extinction of the species such that the species warrants listing as endangered or threatened as those terms are defined by the Act. This does not necessarily require empirical proof of a threat. The combination of exposure and some corroborating evidence of how the species is likely impacted could suffice. The mere identification of factors that could impact a species negatively is not sufficient to compel a finding that listing is appropriate; we require evidence that these factors are operative threats that act on the species to the point that the species meets the definition of an endangered or threatened species under the Act.
In making our revised 12-month finding on the petition, we consider and evaluate the best available scientific and commercial information. This evaluation includes all factors we previously considered in the 2010 finding and, at the end of this analysis, explains how the Services’ conclusions differ now.
Factor A. The Present or Threatened Destruction, Modification, or Curtailment of Its Habitat or Range
Curtailment of Range and Distribution
The range and distribution of fluvial Arctic grayling in the upper Missouri River basin was reduced over the past 100 years (Kaya 1992, p. 51), primarily due to historical habitat fragmentation by dams and irrigation diversions and by habitat degradation or modification from unregulated land use (Vincent 1962, pp. 97-121). Fluvial Arctic grayling typically need large expanses of connected habitat to fulfill their life-history stages (Armstrong 1986, p. 8). For example, fluvial Arctic grayling in the Big Hole River have been documented migrating over 60 miles (97 km) between overwintering, spawning, and foraging habitats (Shepard and Oswald 1989, pp. 18-21, 27). These past reductions in range and distribution reproductively isolated fluvial Arctic grayling populations within the basin (Peterson and Ardren 2009, p. 1770).
Although the range and distribution of fluvial Arctic grayling has contracted from historical levels, expression of the fluvial life history is represented, at least in part, in four Arctic grayling populations within the Upper Missouri River DPS. Whether strictly fluvial (e.g., Big Hole and Ruby River) or partially fluvial (e.g., Centennial Valley (Long Creek) and Ennis Reservoir/Madison River (mainstem Madison River)), these populations occur in four watersheds where large reaches of connected habitat remain and still permit the expression of the fluvial life history, despite the presence of mainstem dams in three of four watersheds (Kaya 1992, entire; see Figure 1). Thus, despite historical curtailment of range, the amount of connected habitat in some systems is adequate to permit the expression of the fluvial life history.
Of the four Arctic grayling populations still expressing a fluvial life history, three of four populations (Big Hole River, Centennial Valley, and Ruby River) are currently increasing in abundance (see Table 3). In each of these populations, as abundance increases, there is a corresponding increase in distribution. Natural reproduction is occurring in all three of these populations. In the Big Hole River and the Centennial Valley, remote site incubators (RSIs) have been used as a conservation tool to help facilitate increased abundance and distribution of Arctic grayling. Thus, observed increases in abundance and distribution may be partially attributable to the use of RSIs (for more in-depth discussion on RSI use, see “Native Arctic Grayling Genetic Reserves and Translocation,” below). Given the above information, it appears that three of four fluvial, or partly fluvial, populations are viable and have the necessary configuration and amount of habitat to fulfill their life-history needs. Thus, effects of past range curtailment on the fluvial component of Arctic grayling in the upper Missouri River basin are present, but there appears to be sufficient adequate habitat remaining to support expression of the fluvial life history.
Adfluvial Arctic grayling populations in the upper Missouri River basin are present in all lakes originally thought to have had native populations historically (Miner, Mussigbrod, Upper Red Rock, and Elk Lakes (present but not included in Table 3, above, because of uncertain viability)). Thus, there has been no contraction of the range of adfluvial populations. Given the above information, curtailment of range and distribution is not precluding the expression of either fluvial or adfluvial life history. Although curtailment of range and distribution occurred historically, Arctic grayling populations are still present in 7 of 10 historically occupied watersheds in the upper Missouri River basin (see “Drainage” column in Table 3). Accordingly, we have no evidence that curtailment of range and distribution is a current threat to the DPS. In addition, we have no information suggesting curtailment of range and distribution will be a threat in the future.
Dams on Mainstem Rivers
Much of the historical range of the Upper Missouri River DPS of Arctic grayling has been altered by the construction of dams and reservoirs (Kaya 1990, pp. 51-52; Kaya 1992, p. 57). The construction of large dams on mainstem river habitats throughout the upper Missouri River system fragmented river corridors necessary for the expression of Arctic grayling migratory life histories in some systems. Construction of dams that obstructed fish passage on the mainstem Missouri River (Hauser, Holter, Canyon Ferry, and Toston dams), Madison River (Madison-Ennis, Hebgen dams), Beaverhead River and its tributary Red Rock River (Clark Canyon, Lima dams), Ruby River (Ruby dam), and Sun River (Gibson dam) all likely contributed to the historical decline of fluvial Arctic grayling in the DPS (Vincent 1962, pp. 127-128; Kaya 1992, p. 57). Lack of fish passage at these dams contributed to the extirpation of fluvial Arctic grayling from some waters by blocking migratory corridors (Vincent 1962, p. 128), curtailing access to important spawning and rearing habitats, and impounding water over former spawning locations (Vincent 1962, p. 128). Most dams within the upper Missouri River basin were constructed between 1905 and 1960 (Kaya 1990, entire).
Despite the construction of multiple dams throughout the historical range of Arctic grayling, multiple populations, or portions of populations, of the fluvial ecotype are still represented in the DPS. These populations reside in areas where sufficient quantity and quality of habitat exist and permit the expression of this life history. In some cases, dams may be providing a benefit, because currently many of the dams that historically affected fluvial Arctic grayling populations are now precluding invasion by nonnative fish from downstream sources. For example, Lima Dam in the Centennial Valley is currently precluding brown trout invasion from downstream sources (Mogen 2014, pers. comm). Currently, there are five Arctic grayling populations within the DPS that occur above mainstem dams (Centennial Valley, Ruby River, Hyalite Lake, Diversion Lake, and Gibson Reservoir) with at least one nonnative fish species occurring downstream of these dams (MFISH 2014d, unpublished data).
Some reservoirs created by dams are currently being used by Arctic grayling as overwintering, rearing and foraging areas. Both adult and juvenile Arctic grayling use Ennis Reservoir for overwintering, rearing, and foraging (Byorth and Shepard 1990, entire). In the Centennial Valley, Arctic grayling have recently been detected in Lima Reservoir (MFISH 2014e, unpublished data). The movements of Arctic grayling within and out of Lima Reservoir are unknown; however, Lima Reservoir is a large reservoir and, as such, is likely used for overwintering purposes.
Arctic grayling have been documented in stream and river reaches below some dams, most likely indicating downstream passage of fish over or through dams. These fish are essentially “lost” to the population residing above the dam, because none of the mainstem river dams in the upper Missouri River basin provides upstream fish passage. Substantial losses from a population resulting from downstream entrainment of fish through dams could cause declines in reproductive potential and abundance in the reservoir population above the dam (Kimmerer 2008, entire). However, it is unknown what entrainment rates currently are in populations residing near dams. Rate of entrainment is likely dependent on a number of factors, including dam operations, season, water conditions in the reservoir, initial population size above the dam, etc. Recent monitoring data and angler reports of Arctic grayling observed downstream of reservoirs supporting Arctic grayling populations are sporadic (Horton 2014c, pers. comm.; SSA 2014); thus it appears the threat of mainstem dams is likely affecting some individuals, but not affecting populations or the DPS as a whole.
Historically, operational practices at Madison Dam have likely affected the Arctic grayling population in Ennis Reservoir/Madison River. A population decline in Arctic grayling appeared to coincide with a reservoir drawdown in the winter of 1982-1983 (Byorth and Shepard 1990, pp. 52-53). This drawdown likely affected the forage base, rearing habitat, and spawning cycle of Arctic grayling in the reservoir. However, under a new licensing agreement dated September 27, 2000, between the Federal Energy Regulatory Commission and Ennis Dam operators, such substantial drawdowns in elevation of Ennis Reservoir are no longer permitted (Clancey 2014, pers. comm.).
Given the above information, mainstem dams were a historical threat to Arctic grayling populations in the upper Missouri River basin. Dams still impact individuals, because some Arctic grayling are currently being entrained and lost from their source population. In Ennis Reservoir, the new licensing agreement is expected to reduce the effects of dam operations on the Arctic grayling population. Most Arctic grayling populations residing above dams are stable or increasing; thus, it does not appear this impact is acting at the population or DPS level. We have no information to conclude that mainstem dams will be a threat in the future at the population or DPS level.
Water Management in the Upper Missouri River Basin
The predominant use of private lands in the upper Missouri River basin is irrigated agriculture and ranching. These activities have historically had significant effects on aquatic habitats, primarily changes in water availability and alteration of the structure and function of aquatic habitats. Changes in water availability can affect Arctic grayling reproduction, survival, and movements among habitat types (Kaya 1990, entire).
In contrast to most of the Arctic grayling populations in the Upper Missouri River DPS that occur on Federal land, the fluvial population of Arctic grayling in the Big Hole River occurs on primarily (∼90 percent) private land. Thus, any conservation efforts conducted in the Big Hole River Valley need support from involved agencies and private landowners. In 2006, a candidate conservation agreement with assurances (CCAA; Montana Fish, Wildlife, and Parks et al. 2006, entire) was developed for Arctic grayling in the Big Hole River. The conservation goal of this CCAA is to secure and enhance the fluvial population of Arctic grayling in the upper Big Hole River drainage. Conservation projects conducted under the CCAA are prioritized and guided by the Big Hole Arctic Grayling Strategic Habitat Conservation Plan (SHCP) (for more specific information, see “Conservation Efforts to Reduce Habitat Destruction, Modification, or Curtailment of Its Range,” below).
Since 2006, many conservation and restoration projects have been completed in the upper Big Hole River under the direction of the CCAA and SHCP (Table 4). Below, we describe and evaluate the implementation and effectiveness of these projects relative to the potential stressors analyzed under Factor A for the Big Hole River population. We also analyze the effects of potential stressors under Factor A for the other Arctic grayling populations in the DPS.
Threat factor | Stressor | Conservation projectsa | Conservation result | Arctic grayling response |
---|---|---|---|---|
[All information on conservation projects and conservation results cited from the Big Hole Arctic Grayling Strategic Habitat Conservation Plan] | ||||
aPODs = Points of Diversion, SSPs = Site-specific plans;bTier I is core spawning, rearing and adult habitat that is currently occupied by Arctic grayling, Tier II is periphery habitat intermittently used by Arctic grayling, Tier III is suitable, but currently unoccupied historical habitat;cThe estimate of number of breeding adults in the Big Hole River in 2013 is reported as a range because of uncertainty in the frequency rate of rare alleles in the analysis;dAbundance estimates from 2013 were lower than those reported for 2012 likely due to unusually high flows (3X normal) concurrent with fall sampling that likely decreased capture efficiency, resulting in lower abundance estimates in 2013. | ||||
A | Dams/habitat fragmentation | Fish ladders: 41 Bridges: 7 Grade control structures: 2 | Stream miles (%) accessible to graylingb: • Tier I- 82(98%; pre-CCAA=87%). • Tier II- 61(67%; pre-CCAA=27%). • Tier III- 32(20%: pre-CCAA=6%). | • Number of breeding adults has increased from ∼100 (2007-2011) to 500-900c(2013) (Leary 2014, unpublished data). |
Dewatering/Thermal stress | PODs: 343 of 504 with signed SSPs Irrigation improvements: 88 Water measuring devices: 67 Stock water systems: 63 Stream restoration: 26 miles Rock Creek restoration | • Achievement of instream flow goals increased from 50% (pre-CCAA) to 78% (post-CCAA) • Landowner contributions to streamflow increasing as # of PODs with signed SSPs increase [landowner contribution to instream flows in Big Hole River (pre-2006 = 0 cfs; 2013 = 250 cfs)] • Temperature reductions in tributaries (see Rock Creek example below) | • Arctic grayling abundanced(catch per unit effort) increased from 0.2 fish/mile (2008) to 1.4 fish/mile (2012) in the CCAA monitoring reaches of the mainstem Big Hole River (MFWP 2013a, unpublished data). | |
Pre-restoration (2007): • 36 days max. temp >70 °F • 16 days max. temp >77 °F Post-restoration (2013): • 0 days max temp. >70 °F | • Arctic grayling abundanced(catch per unit effort) increased from 2.9 fish/mile (2008) to 7.4 fish/mile (2012) in the CCAA monitoring tributaries (MFWP 2013a, unpublished data). • Arctic grayling distribution has increased 4 miles in Rock Creek (young-of-year and Age 1+) and 2 miles in Big Lake Creek (Age 1+) since 2006 (SHCP 2013, p. 12). | |||
Entrainment | Fish screens: 2 Prioritized monitoring protocol | • No entrainment documented since 2010 • Observed low entrainment rates in unscreened ditches (73 Arctic grayling/138 ditch miles) | ||
Riparian habitat loss | Stream restoration: 26 miles Riparian fencing: 108 miles Stock water systems: 63 Grazing mgmt. plans: 21 landowners (85,000 ac.) Noxious weed management Willow planting (72,200 planted) | • 110 miles (65%) of riparian habitat on enrolled lands improving • 15% increase in sustainable riparian areas from 32% (2006) to 47% (2013) • Adaptive management in place to address non-improving areas |
Habitat Fragmentation/Smaller Seasonal Barriers
Big Hole River: Smaller dams or diversions associated with irrigation structures historically posed a threat to Arctic grayling migratory behavior, especially in the Big Hole River drainage. In the Big Hole River, numerous diversion structures have been identified as putative fish migration barriers (Petersen and Lamothe 2006, pp. 8, 12-13, 29) that may limit the ability of Arctic grayling to migrate to spawning, rearing, or sheltering habitats under certain conditions. As with the larger dams, these smaller fish passage barriers can reduce reproduction (access to spawning habitat is blocked), reduce growth (access to feeding habitat is blocked), and increase mortality (access to refuge habitat is blocked). Historically, these types of barriers were numerous and widespread across the Big Hole River drainage.
Currently, habitat fragmentation due to irrigation diversion structures in the Big Hole is being systematically reduced under the CCAA for Fluvial Arctic Grayling in the upper Big Hole River (hereafter, Big Hole CCAA or CCAA; for more specific information, see “Conservation Efforts to Reduce Habitat Destruction, Modification, or Curtailment of Its Range”) and Big Hole Arctic Grayling SHCP. Since 2006, 41 fish ladders have been installed in the mainstem Big Hole River and tributaries (Table 4). Multiple culverts have been replaced with bridges and several grade control structures have been installed (Table 4). As a result, no fish barriers now exist in the mainstem upper Big Hole River. Almost all (98 percent) of tier I habitat and the majority (68 percent) of tier II habitat is connected and accessible to Arctic grayling (Table 4): 67 miles of stream have been reconnected in the Big Hole River system since 2006 (MFWP 2014a, unpublished data).
Other populations: Smaller fish passage barriers also have been noted to affect Arctic grayling in the Centennial Valley (Unthank 1989, p. 9). Historically, spawning Arctic grayling migrated from the Jefferson River system, through the Beaverhead River and Red Rock River through the Red Rock Lakes and into the upper drainage, and then returned downstream after spawning (Henshall 1907, p. 5). The construction of a water control structure (sill) at the outlet of Lower Red Rock Lake in 1930 (and reconstruction in 1957 (USFWS 2009, p. 74)) created an upstream migration barrier that blocked these migrations (Unthank 1989, p. 10; Gillin 2001, p. 4-4). However, recent changes in water management at the Red Rock Lakes National Wildlife Refuge (NWR) have resulted in year-round fish passage through the control structure at the outlet of Lower Red Rock Lake (West 2013, pers. comm.).
In Mussigbrod Lake, Arctic grayling occasionally pass downstream over a diversion structure at the lake outlet, and become trapped in an isolated pool (Olsen 2014, pers. comm.). During high-snowpack years, Arctic grayling likely can swim back up to the lake from the pool, but in low snowpack years, some Arctic grayling perish when the isolated pool dries up (Olsen 2014, pers. comm.). However, this phenomenon has occurred periodically in recent history and has had no discernible impacts on Arctic grayling abundance in Mussigbrod Lake (Olsen 2014, pers. comm.).
All 16 adfluvial Arctic grayling populations in the upper Missouri River basin occur on Federal land (U.S. Forest Service) and are not influenced by irrigation structures because none are present. The effect of a barrier at the outlet of Mussigbrod Lake is likely impacting individuals, but not the population because of the robust population size in Mussigbrod Lake and historical stability of that population since the outlet structure was created. Based on this information, we conclude that the threats from habitat fragmentation have been sufficiently mitigated or minimized and are no longer are acting as a stressor at the population or DPS level.
Degradation of Riparian Habitat
Riparian corridors are important for maintaining habitat for Arctic grayling in the upper Missouri River basin, and in general are critical for the ecological function of aquatic systems (Gregory et al. 1991, entire). Riparian zones are important for Arctic grayling because of their effect on water quality and water temperature, and their role in maintaining natural ecological process responsible for creating and maintaining necessary physical habitat features (i.e., pools, riffles, and scour areas) used by the species to meet its life-history requirements.
Big Hole: Arctic grayling abundance in the upper Big Hole River is positively related to the presence of overhanging vegetation, primarily willows (Salix spp.), that is associated with pool habitat (Lamothe and Magee 2004, pp. 21-22). Removal of willows and riparian clearing concurrent with livestock and water management along the upper Big Hole River has led to a shift in channel form (i.e., braided channels becoming a single wide channel), increased erosion rates, reduced cover, increased water temperatures, and reduced recruitment of large wood debris into the active stream channel (Confluence Consulting et al. 2003, pp. 24-26). These factors combine to reduce the suitability of the habitat for species like Arctic grayling (Hubert 1985, entire).
Currently, restoration of riparian areas in the upper Big Hole River system is a priority under the CCAA (for more specific information, see “Conservation Efforts to Reduce Habitat Destruction, Modification, or Curtailment of Its Range,” below). Since 2006, efforts to restore and conserve riparian habitats have been numerous and multi-faceted (see Table 4). About 170 miles (274 km) of riparian habitat are currently enrolled in the Big Hole CCAA, out of a total of about 340 miles (547 km) of total riparian habitat in the CCAA Management Area. Of the enrolled riparian habitat, 65 percent (110 miles (177 km)) is improving in condition, as rated by a standardized riparian protocol (NRCS 2004, entire). Further, 47 percent of enrolled riparian habitat (80 miles (129 km)) is functioning at a sustainable level, which is a 15 percent increase in 5 years (MTFWP et al. 2006, p. 92; see Table 4). A sustainable rating indicates that the stream can access its flood plain, transport its sediment load, build banks, store water, and dissipate flood energy in conjunction with a healthy riparian zone (NRCS 2004, p. 7). Riparian habitats are reassessed every 5 years and are scored on 10 stability and sustainability metrics (for example, stream incisement), with any reach scoring at 80 percent or above rated as sustainable (NRCS 2004, entire). In addition, adaptive management within the CCAA framework will allow for reevaluation of conservation measures being implemented in non-improving habitat.
Other populations: In the Centennial Valley, historical livestock grazing both within the Red Rock Lakes NWR and on adjacent private lands negatively affected the condition of riparian habitats on tributaries to the Red Rock Lakes (Mogen 1996, pp. 75-77; Gillin 2001, pp. 3-12, 3-14). In general, degraded riparian habitat limits the creation and maintenance of aquatic habitats, especially pools, which are preferred habitats for adult Arctic grayling (Lamothe and Magee 2004, pp. 21-22; Hughes 1992, entire), although many spawning adult Arctic grayling in Red Rock Creek outmigrate soon after spawning and likely do not use available pool habitat (Jordan 2014, pers. comm.). Loss of riparian vegetation increases bank erosion, which can lead to siltation of spawning gravels, which may in turn harm Arctic grayling by reducing the extent of suitable spawning habitat and reducing survival of Arctic grayling embryos already present in the stream gravels.
Recently, the Red Rock Lakes NWR acquired land on Red Rock Creek, upstream of the refuge boundary (West 2014a, pers. comm.). Much of this parcel was riparian habitat that was historically heavily grazed; thus, the refuge implemented a rest-rotation grazing system where more durable lands are grazed while more sensitive lands (e.g., riparian areas) are rested for up to 4 years. On average, grazing intensities on the refuge have decreased from 20,000 Animal Unit Months (AUMs, number of cow/calf pairs multiplied by the number of months grazed) to about 5,000 AUMs. As a result of these changes, riparian habitat within the refuge has dramatically improved (West 2014b, pers. comm.) and is expected to continue improving under the new grazing regime. Concurrent with riparian improvement within Red Rock Lakes NWR, the number of adult Arctic grayling migrating up Red Rock Creek to spawn has increased from fewer than 500 to more than 2,000 (Patterson 2014, unpublished data). Given the riparian improvements within Red Rock Lakes NWR, and that the refuge represents the vast majority of current Arctic grayling habitat in the Centennial Valley, the effects of degraded riparian habitat do not appear to be acting on the core of the Centennial Valley population at the individual or population level.
Most of the riparian habitat surrounding high-elevation lakes on Federal land where the remaining populations are found is intact and of high quality (MFISH 2014a, unpublished data; MFWP 2014e, unpublished data; USFS 2014, p. 2), because these habitats are in remote locations or wilderness areas with little anthropogenic disturbance. Given that riparian degradation is being systematically addressed in the Big Hole River and Centennial Valley on the National Refuge land where the majority of Arctic grayling reside, we conclude that riparian degradation is not a current threat to the DPS. Riparian habitat is expected to remain intact on Federal land because of existing regulatory mechanisms (see in Factor D discussion, below). Riparian habitat in the Big Hole River is expected to continue improving because of the proven track record of conservation evidenced by the current upward trend in riparian habitat quality. As more site-specific plans are signed under the Big Hole CCAA, more riparian improvement is expected because conservation measures will be similar between currently implemented and future site-specific plans. Given that riparian habitat is intact or improving for populations of Arctic grayling occurring on Federal land and the Big Hole population, and these populations account for 19 of 20 populations in the DPS, we conclude riparian habitat degradation is not a current rangewide threat and is not expected to become a threat in the future.
Dewatering From Irrigation and Consequent Increased Water Temperatures
Demand for irrigation water in the semi-arid upper Missouri River basin historically dewatered many rivers formerly or currently occupied by Arctic grayling. The primary effects of this dewatering were: (1) Increased water temperatures, and (2) reduced habitat capacity. In ectothermic species like salmonid fishes, water temperature sets basic constraints on species’ distribution and physiological performance, such as activity and growth (Coutant 1999, pp. 32-52). Increased water temperatures can reduce the growth and survival of Arctic grayling (physiological stressor). Reduced habitat capacity can concentrate fishes and thereby increase competition and predation (ecological stressor). Below we discuss the potential effects of increased water temperature on the Upper Missouri River DPS of Arctic grayling. For discussion of the potential effects of reduced habitat capacity, see Cumulative Effects from Factors A through E, below.
Big Hole: In the Big Hole River system, surface-water (flood) irrigation has altered the natural hydrologic function of the river (Shepard and Oswald 1989, p. 29; Byorth 1993, p. 14; 1995, pp. 8-10; Magee et al. 2005, pp. 13-15). An inverse relationship between flow volume and water temperature (i.e., lower flows can lead to higher water temperatures) is apparent in the Big Hole River (Flynn et al. 2008, pp. 44, 46, but see Sladek 2013, p. 31). Summer water temperatures exceeding 21 °C (70 °F) are considered to be physiologically stressful for cold-water fish species, such as Arctic grayling (Hubert et al. 1985, pp. 7, 9). Summer water temperatures consistently exceed 21 °C (70 °F) in the mainstem of Big Hole River (Cayer and McCullough 2012, p. 7; (Cayer and McCullough 2013, p. 6) and have exceeded the upper incipient lethal temperature (UILT; the temperature that is survivable for periods longer than 1 week by 50 percent of a “test population” in an experimental setting) for Arctic grayling (e.g., 25 °C or 77 °F) (Lohr et al. 1996). As a result, thermal fish kills have been documented in the Big Hole River (Lohr et al. 1996, p. 934) in the past. The most recent fish kill in the Big Hole River that we are aware of occurred in 1994, and included eight fish species, including Arctic grayling (Lohr et al. 1996, p. 934).
Arctic grayling in the Big Hole River use tributaries as a thermal refuge when summer water temperatures in the mainstem become stressful (Vatland et al. 2009, p. 11). Summer water temperatures within most tributaries are cooler than those observed in some reaches of the mainstem Big Hole River (Vatland et al. 2009, entire; MFWP 2014b, unpublished data).
Since 2006, water conservation and restoration projects associated with the Big Hole Arctic grayling CCAA (for more specific information, see “Conservation Efforts to Reduce Habitat Destruction, Modification, or Curtailment of Its Range,” below) have been implemented to increase instream flows and reduce water temperatures in the Big Hole River and tributaries. Varying flow targets for different management segments of the Big Hole River were outlined in the CCAA, based on the wetted perimeter method, a biologically based method for determining instream flow requirements to provide necessary resources for all life stages of Arctic grayling. Over 300 irrigation diversions are operated under flow agreements within finalized site-specific plans (Table 4). The 10 remaining site-specific plans representing the remainder of points of diversion are expected to be signed in August 2014. Although we are aware of the future potential of more points of diversion being managed under signed site plans to contribute to Arctic grayling conservation, we do not consider these anticipated future efforts to contribute to Arctic grayling conservation currently, and have not considered them as part of this status review or our listing determination for this DPS. Multiple other projects designed to decrease dewatering and thermal stress have been implemented since 2006 (Table 4). The collective result of these efforts are increasing streamflows, increased access to cold-water refugia via fish ladders, and marked temperature reductions, particularly in some tributaries (Table 4).
Specific flow targets were developed for the different Management Segments in the CCAA Management Area (see MFWP et al. 2006, pp. 7, 9, 13, for more information on CCAA Management Segments). The goal for increasing instream flow was to achieve flow targets 75 percent of days in each Management Segment during years of average or greater snowpack. This goal was based on a comparison between minimum flow targets and historical streamflows recorded in Management Segments C and D. Achieving flow targets 75 percent of days in each Management Segment was intended to be a general goal because many other factors influence instream flows in the Big Hole River that are outside the control of landowners (e.g., snowpack, precipitation). Before implementation of the CCAA (2000-2005), average flow targets were met among all Management Segments 50 percent of the time, and since implementation of the CCAA (2006-2012), they have been met 78 percent of the time (SHCP 2013, p. 12). Thus, the targets are being met.
Consistently since 2006, one management area, known as Management Segment C, has exhibited the lowest instream flows among all Management Segments. In part, instream flows in Management Segment C are influenced by several large diversions immediately upstream of the flow measuring device at the downstream boundary of Management Segment C (Robert 2014, pers. comm.). Some of this diverted water is returned to the Big Hole River downstream of the flow measuring device (Robert 2014, pers. comm.). As such, instream flows in Management Segment C represent the “worst case” scenario among all Management Segments. The Montana Department of Natural Resources and Conservation conducted an analysis of this “worst case” scenario, to explore how instream flows in Management Segment C have changed since the inception of the Big Hole CCAA. Given that natural factors such as summer precipitation and annual snowpack influence instream flows in the Big Hole River, the analysis of instream flows in Management Segment C included comparisons among several years of similar (but below average) snow pack and similar summer precipitation, both before and after CCAA implementation (Table 5).
Pre-CCAA | Post-CCAA | |||
---|---|---|---|---|
1988 | 2003 | 2012 | 2013 | |
aNormalized to base period 1971-2000;b160 cfs = flow target for Spring (April-June), 60 cfs = flow target for Summer and Fall (July-October) in CCAA Management Area C; Cfs = cubic feet per second. | ||||
Peak snowpack (percent of average) | a73 | 108 | 81 | a75 |
May-Aug. precipitation (in.) | 4.14 | 3.85 | 4.74 | 5.14 |
July-Aug. temps (degrees F; departure from normal) | −1.3 | 8.0 | 1.4 | 1.9 |
Signed SSPs | 0 | 0 | 12 | 15 |
Landowner contributions (cfs) | 0 | 0 | 252 | 260 |
Days <160bcfs | 50 | 8 | 11 | 40 |
Days <60bcfs | 123 | 123 | 87 | 69 |
Days <20 cfs | 79 | 68 | 0 | 28 |
Days <10 cfs | 65 | 7 | 0 | 1 |
Mean discharge (cfs; July-Sept.) | 8.4 | 19.7 | 45 | 39 |
Mean discharge (cfs; Aug.) | 1.1 | 14.2 | 33.7 | 21 |
Total Days <Streamflow target | 173 | 131 | 98 | 109 |
In pre- and post-CCAA years, flows in Management Segment C were less than the Spring flow target of 160 cfs (14.5 cubic meters/second (m3/s)) for similar numbers of days (Table 5). However, number of days where flows were less than the Summer/Fall flow target of 60 cfs (1.7m3/s) decreased 37 percent pre- to post-CCAA (average of 123 days (pre-CCAA) to 78 days (post-CCAA)). Number of days instream flows were below 20 and 10 cfs (0.6 and 0.3 m3/s, respectively) (these flows represent common, historical low water levels in Management Segment C) were reduced 81 percent, and 99 percent, respectively, from pre- to post-CCAA implementation (Table 5). In brief, there has been an observed 300 percent increase in average discharge from July through September (from 14 to 42 cfs (0.4 and 1.2 m3/s, respectively)) as a result of achieving flow targets in Management Area C for a higher percentage of days post-CCAA, relative to pre-CCAA (Roberts 2014, unpublished data). Landowner contributions to instream flow from reducing irrigation withdrawals appears to be the primary factor increasing instream flows in the Big Hole River in late summer (Table 5), a critical thermal period for Arctic grayling.
Despite Management Segment C exhibiting the lowest rate of instream flow achievement relative to the other Management Segments, we note that the proportion of Tier I habitat encompassed by Management Segment C is 12 percent; the remainder of Tier I habitat (88 percent) is located in Management Segments D and E (MFWP 2014c, unpublished data). Since the initiation of the Big Hole Arctic grayling CCAA in 2006, average achievement rate of instream flow goals in Management Segments D and E during the spring is 96 percent and 99 percent, respectively. Average achievement rate of instream flow goals in Management Segments D and E during the summer/fall is 84 percent and 76 percent, respectively. Thus, flow targets are being met. We conclude the Big Hole CCAA has ameliorated dewatering as a stressor in the Big Hole River.
Other populations: Increased water temperatures also are present in the Madison River and Centennial Valley. Mean and maximum summer water temperatures can exceed 21 °C (70 °F) in the Madison River below Ennis Reservoir (U.S. Geological Survey (USGS) 2010), and have exceeded 22 °C (72 °F) in the reservoir, and 24 °C (75 °F) in the reservoir inlet (Clancey and Lohrenz 2005, p. 34). However, Arctic grayling in these systems appear to be able to cope with these temperatures by using cooler tributaries and spring sources as thermal refugia (Jaeger 2014b, pers. comm.). For example, the presence of Arctic grayling in the lower 100 m (328 ft) of East Shambow Creek in 1994 was attributed to fish seeking refuge from high water temperatures in the lake (Mogen 1996, p. 44). The Centennial Valley, in particular, appears to have many cool spring-fed tributaries that are accessible to Arctic grayling and are used intermittently (Mogen 1996, p. 44). Mean summer water temperatures in Red Rock Creek can occasionally exceed 20 °C (68 °F) during drought conditions (Mogen 1996, pp. 19, 45); however, on average, these are much cooler than summer water temperatures observed in the Big Hole River.
Increased water temperatures do not appear to be prevalent in most other adfluvial populations, likely due to the high elevation of these habitats and the intact nature of riparian areas bordering inlet tributaries. Given the presence of cooler tributaries and spring sources used by Arctic grayling in the Centennial Valley and Ennis Reservoir/Madison River, it does not appear thermal stress is a current threat to these populations. Although water temperatures will likely increase with climate change in the future, the spring-fed sources of cool water will likely remain intact and within a temperature range suitable for Arctic grayling occupancy. Thus, thermal stress is not expected to be a future threat.
Entrainment
Entrainment can permanently remove individual fish from a natural population and strand them in a habitat that lacks the required characteristics for reproduction and survival. Irrigation ditches may dry completely when irrigation headgates are closed, resulting in mortality of entrained Arctic grayling.
Big Hole: Entrainment of individual Arctic grayling in irrigation ditches historically occurred and currently occurs in the Big Hole River (Skarr 1989, p. 19; Streu 1990, pp. 24-25; MFWP et al. 2006, p. 49; Lamothe 2008, p. 22; MFWP 2013b, unpublished data). Over 1,000 unscreened diversion structures occur in the upper Big Hole River watershed, and more than 300 of these are located in or near occupied Arctic grayling habitat (MFWP et al. 2006, pp. 48-49).
However, recent entrainment surveys in irrigation ditches along the mainstem Big Hole River and tributaries indicate low levels of Arctic grayling entrainment. Since 2006, 138 ditch miles have been sampled using electrofishing to estimate entrainment, resulting in the capture of 73 Arctic grayling, most of which were young-of-year (MFWP 2013b, unpublished data). This number is very low relative to the size of the population. All documented entrainment has occurred in 4 irrigation ditches, one of which recently had a fish screen installed (MFWP 2013b, unpublished data). No entrainment of Arctic grayling has been documented in any irrigation ditch since 2010 (including the 4 previously mentioned where entrainment of Arctic grayling had occurred), despite intensive sampling by Montana Fish, Wildlife, and Parks (Cayer 2014b, pers. comm.). We do note that sampling typically does not occur during the larval stage for Arctic grayling. Larval losses into irrigation ditches could be substantial and go undetected under the current sampling protocol. However, observations of young of year Arctic grayling in the Big Hole River indicate that many, but not likely all, newly emerged fry stay relatively close to the area where they were born (Skaar 1989, p. 51; Streu 1990, p. 28; McMichael 1990, p. 38), thus reducing the risk of entrainment because of minimal instream movements during their first summer.
Irrigation ditches are prioritized and systematically monitored based on the ditch location relative to known Arctic grayling distribution, additive maximum flow rate, and distance from the mainstem Big Hole River (MFWP et al. 2006, p. 116). In addition, electrofishing efficiency in simple habitats (such as irrigation ditches) is high (Kruse et al. 1998, pp. 942-943); thus, we have high confidence that these surveys have been accurate and that entrainment in the Big Hole River system is currently low and likely not a threat at the population level.
Other populations: Entrainment was likely a historical threat for Arctic grayling at some locations within the Centennial Valley (Unthank 1989, p. 10; Gillin 2001, pp. 2-4, 3-18, 3-25), particularly outside of the Red Rock Lakes NWR (Boltz 2010, pers. comm.). Currently, one irrigation ditch is present near the core of the Centennial Valley population within the Red Rock Lakes NWR. This ditch conveys water from Red Rock Creek to a waterfowl slough for a portion of the year; however, it is not operated by the Refuge when Arctic grayling fry are expected to be in Red Rock Creek (Bill West 2014a, pers. comm.). Other irrigation ditches are present upstream and downstream of the NWR boundary; however, Arctic grayling densities in these areas are low, and any mortality associated with entrainment in these areas is expected to be negligible at the population level.
Entrainment of Arctic grayling does not appear to be a threat in the Big Hole River and Centennial Valley populations. Habitats occupied by all 16 adfluvial Arctic grayling populations in the upper Missouri River basin are not subjected to irrigation withdrawals; thus entrainment is not a threat to these populations. We expect irrigation withdrawal volume to remain similar to current levels, particularly in the Big Hole River, in the future as more flow agreements are signed under the CCAA. Thus, we conclude entrainment will likely not be a future threat.
Sedimentation
Sedimentation has been proposed as a mechanism behind the decline of Arctic grayling and its habitat in the Centennial Valley (Unthank 1989, p. 10; Mogen 1996, p. 76), which includes Upper and Lower Red Rock Lakes. Historically, livestock grazing upstream likely led to accelerated sediment transport in tributary streams, and deposition of silt in both stream and lakes, thus modifying and reducing fish habitat by filling in pools, covering spawning gravels, and reducing water depth in Odell and Red Rock Creeks, where Arctic grayling spawn (MFWP 1981, p. 105; Mogen 1996, pp. 73-76). Sedimentation in the Upper and Lower Red Rock Lakes is believed to affect Arctic grayling in winter by reducing habitat volume (e.g., lakes freezing to the bottom) and promoting hypoxia (low oxygen), which generally concentrates fish in specific locations, thus increasing the probability of competition and predation. In summer, reduced habitat volume could contribute to increased warming.
Dissolved oxygen levels in Upper Red Rock Lake during winter can drop below levels typically considered lethal for Arctic grayling (Gangloff 1996, pp. 41-42, 72). As a result, winter kill of invertebrates and fishes (e.g., suckers (Catostomus spp.)) has been recorded in Upper Red Rock Lake (Gangloff 1996, pp. 39-40); however, no Arctic grayling kills have been documented. Gangloff (1996, pp. 71, 79) hypothesized that Arctic grayling in Upper Red Rock Lake exhibit behavioral mechanisms or physiological adaptations that permit them to survive otherwise lethally low oxygen levels. Arctic grayling under winter ice seek areas of higher oxygen concentration (oxygen refugia) within the lake or near inlet streams of Upper Red Rock Lake (Gangloff 1996, pp. 78-79).
It has been reported that depths in the Red Rock Lakes have decreased significantly, with a decline in maximum depth from 7.6 to 5.0 m (25 to 16.4 ft) to less than 2 m (6.5 ft) noted in Upper Red Rock Lake over the past century (Mogen 1996, p. 76). This conclusion is prevalent among historical accounts of the Centennial Valley. However, a more recent analysis of sedimentation entering Upper Red Rock Lake indicated modest rates of sediment accumulation in Upper Red Rock Lake over the last century and that the rate of infilling in Upper Red Rock Lake has been relatively constant, based on lead and cesium analysis in lake bottom cores (Allison 1996, unpublished data). Thus, it appears historical accounts of rapid infilling of Upper Red Rock Lake were invalid and that sedimentation in Upper Red Rock Lake is not a stressor.
Sedimentation in tributary streams due to unregulated land use may have contributed to historical Arctic grayling declines in the Centennial Valley (Vincent 1962, p. 114). Now, land use is regulated, particularly on Federal land, which comprises the majority of ownership in the Centennial Valley. However, some of the tributary streams in the Centennial Valley are still affected by sediment, even some spring source streams. The effect of these levels of sediment on Arctic grayling in the Centennial Valley is unclear. However, spawning conditions in Red Rock Creek are currently supporting 40-year highs in hybrid cutthroat and Arctic grayling abundance (MFWP 2013c, unpublished data).
The effects of erosion and sedimentation on spawning gravels in Red Rock Creek and reduction of habitat volume in Upper and Lower Red Rock Lakes do not appear to be current threats because improved grazing practices appear to be reducing erosion rates upstream of Red Rock Lakes NWR (USFWS 2009, pp. 75-76; Korb 2010, pers. comm.; West 2014; pers. comm.). Natural infilling of Upper Red Rock Lake is occurring (Allison 1996, unpublished data), but is not occurring at a rate or scale that constitutes a threat to Arctic grayling.
Climate Change
Our analyses under the Endangered Species Act include consideration of ongoing and projected changes in climate. The terms “climate” and “climate change” are defined by the Intergovernmental Panel on Climate Change (IPCC). “Climate” refers to the mean and variability of different types of weather conditions over time, with 30 years being a typical period for such measurements, although shorter or longer periods also may be used (IPCC 2007, p. 78). The term “climate change” thus refers to a change in the mean or variability of one or more measures of climate (e.g., temperature or precipitation) that persists for an extended period, typically decades or longer, whether the change is due to natural variability, human activity, or both (IPCC 2007, p. 78). Various types of changes in climate can have direct or indirect effects on species. These effects may be positive, neutral, or negative and they may change over time, depending on the species and other relevant considerations, such as the effects of interactions of climate with other variables (e.g., habitat fragmentation) (IPCC 2007, pp. 8-14, 18-19). In our analyses, we use our expert judgment to weigh relevant information, including uncertainty, in our consideration of various aspects of climate change.
Water temperature and hydrology (stream flow) are sensitive to climate change, and influence many of the basic physical and biological processes in aquatic systems. For ectothermic organisms like fish, temperature sets basic constraints on species’ distribution and physiological performance, such as activity and growth (Coutant 1999, pp. 32-52). Stream hydrology not only affects the structure of aquatic systems across space and time, but influences the life history and phenology (timing of life-cycle events) of aquatic organisms such as fishes. For example, the timing of snowmelt runoff can be an environmental cue that triggers spawning migrations in salmonid fishes (Brenkman et al. 2001, pp. 981, 984), and the timing of floods relative to spawning and emergence can strongly affect population establishment and persistence (Fausch et al. 2001, pp. 1438, 1450). Significant trends in water temperature and stream flow have been observed in the western United States (Kaushal et al. 2010, entire; Isaak et al. 2012, entire; Null et al. 2013, entire, and climatic forcing (the energy difference between incoming solar radiation and outgoing radiation from Earth) caused by increased air temperatures and changes in precipitation are partially responsible.
Observations on flow timing in the Big Hole River, upper Madison River, and Red Rock Creek in the Centennial Valley indicate a tendency toward earlier snowmelt runoff (Wenger et al. 2011, entire; Towler et al. 2013, entire; De Haan et al. 2014, p. 41). These hydrologic alterations may be biologically significant for Arctic grayling in the Missouri River basin because they typically spawn prior to the peak of snowmelt runoff (Shepard and Oswald 1989, p. 7; Mogen 1996, pp. 22-23; Rens and Magee 2007, pp. 6-7). A trend toward earlier snowmelt runoff has resulted in earlier spawning in European grayling in Switzerland (Wedekind and Kung 2010, pp. 1419-1420). The effects of altered timing of spawn on Arctic grayling demographics are unknown. However, it has been hypothesized that the timing of fry emergence in salmonids is synchronized with when food resources are available (Crozier et al. 2008). Given that many ecological processes in aquatic environments are water temperature dependent (Durance and Ormerod 2007, entire), it is likely that any alterations in timing of salmonid fry emergence would be synchronous with alterations in the timing of emergence and availability of prey species.
Recent climate analyses in the Big Hole River Valley and Centennial Valley indicate rising air temperatures (1.8-3.2 °F (1.0-1.8 °C)/decade) from the 1980s to mid-2000s (De Haan et al. 2014, p. 29). During this time, number of breeding Arctic grayling in the Big Hole River declined while the number of breeding Arctic grayling in the Centennial Valley increased (DeHaan et al. 2014, p. 17), despite a coherent climate signal between both drainages. This may be partially attributable to cool-water springs helping ameliorate increasing air temperatures in the Centennial Valley. Since the late 2000s, the number of breeding Arctic grayling has increased in the Big Hole River (Leary 2014, unpublished data), and the number of Arctic grayling in the Red Rock Creek spawning run has increased in the Centennial Valley (Patterson 2014, unpublished data). Thus, we have no information to conclude that increasing air temperatures have had a significant effect on number of breeding Arctic grayling in these systems in recent years.
The effect of warming water from increased air temperatures would be similar to that described for increased temperatures associated with stream dewatering (see discussion under Factor A), namely there has been an increased frequency of high water temperatures that have the potential to affect survival or optimal growth for Arctic grayling, which is considered a cold-water (stenothermic) species (Selong et al. 2001, p. 1032). However, the transfer of heat from air to water (i.e., convection) is a relatively small proportion of the energy exchange that occurs (Johnson 2003, p. 497). The more important factor influencing water temperature is likely to be solar radiation input (Johnson 2003, p. 497; Cassie 2006, p. 1393). Thus, the changes in ambient air temperature predicted to occur as the climate changes are not likely to have as large an effect on water temperatures as solar radiation. Changes in channel morphology (reducing width-to-depth ratios) and riparian vegetation (shading) resulting from the conservation actions being implemented for Arctic grayling are expected to reduce water temperatures by blocking some solar radiation and reducing surface area that solar radiation can interact with. In the Big Hole River, where riparian areas are improving and braided channels are being restored, substantial reductions in water temperature have been observed (see Table 4, Rock Creek restoration for example). We expect the restoration of riparian areas and concomitant channel morphology changes that have occurred to help mitigate the effects of climate change. In the Centennial Valley, intact riparian areas are expected to mitigate the effects of climate change, through similar processes as in the Big Hole River.
Warming patterns in the western United States are not limited to streams. In California and Nevada, lake water surface temperatures have increased by an average of 0.11 °C (0.2 °F) per year since 1992, and at a rate twice that of the average minimum air surface temperature (Schneider et al. 2009, p. L22402). This suggests lake habitats are not immune to the predicted effects of climate change. Shallow lakes with a large surface area, such as Upper Red Rock Lake and Ennis Reservoir, would be expected to warm faster than deeper lakes. However, all 16 strictly adfluvial Arctic grayling populations in the upper Missouri River drainage occur in lake habitats that are expected to have thermal regimes well below upper thermal tolerances for Arctic grayling because of high elevation, bathymetry (underwater topography), and cool inputs from shaded inlet streams.
The land area of the upper Missouri River basin is predicted to warm through the end of the century (Ray et al. 2010, p. 23), although currently occupied Arctic grayling habitat tends to be in colder areas of moderate-to-high elevation. Most of the Arctic grayling populations are at approximately 1,775 to 2,125 m (5,860 to 9,000 ft) elevation (Peterson and Ardren 2009, p. 1761; MFISH 2014a, unpublished data). Alterations to instream flow and timing of runoff are already documented. However, Arctic grayling are likely to persist in the upper Missouri River drainage because of what appears to be an inherent ability possessed by Arctic grayling to adjust spawn timing with changing water temperature regimes (Wedekind and Kung 2010, pp. 1419-1420). In addition, it has been demonstrated in the Big Hole River and Centennial Valley that Arctic grayling are capable of increasing in abundance and distribution, despite a warming climate (Dehaan et al. 2014, p. 17; Leary 2014, unpublished data). It appears Arctic grayling within the upper Missouri River basin are responding favorably to increasing quality of habitat based on increasing abundance and distribution in systems with large-scale, ongoing habitat improvements (Big Hole River and Centennial Valley). Riparian restoration, particularly in the Big Hole River and Centennial Valley, is expected to minimize the effects of increasing water temperatures due to climate change. Sixteen other adfluvial populations are currently in habitats that will likely not be affected significantly by climate change due to their high elevation, intact riparian areas, and cool inputs of tributary water. Thus, we do not consider climate change to be a current threat to Arctic grayling in the upper Missouri River. Further, observed water temperature reductions from riparian restoration projects indicate that intact riparian areas can mitigate for many of the anticipated effects of climate change in the future. Therefore, we conclude climate change is not a future threat to the Upper Missouri River DPS of Arctic grayling.
Conservation Efforts To Reduce Habitat Destruction, Modification, or Curtailment of Its Range
Big Hole River CCAA
In 2006, a CCAA was developed for Arctic grayling in the Big Hole River. The conservation goal of this CCAA is to secure and enhance the fluvial population of Arctic grayling in the upper Big Hole River drainage. The CCAA Management Area encompasses about 382,000 acres and is divided into five management segments to make the conservation guidelines more spatially meaningful to property owners enrolled in the CCAA and to allow the involved agencies to track the progress of the conservation measures both temporally and spatially.
Site-specific plans are developed with each enrolled landowner; these plans identify conservation actions needed (or already completed) to meet the conservation goals of the CCAA. The conservation guidelines of the CCAA are met by implementing conservation measures that:
(1) Remove barriers to Arctic grayling migration;
(2) Improve streamflows;
(3) Identify and reduce or eliminate entrainment threats for Arctic grayling; and
(4) Improve and protect the function of riparian habitats.
Currently, 31 landowners have enrolled 158,000 acres (∼52 percent total enrollable land) in the Big Hole CCAA Management Area. Of the 31 landowners, 21 have signed (finalized) site-specific plans. The remaining 10 landowners have site-specific plans in various stages of completion. All of these unfinished site plans are scheduled to be finalized by August 2014, and will include measures identified previously in the CCAA that have a successful track record; however, we have not considered future anticipated conservation actions outlined in unsigned site plans as part of this status review or our listing determination for this DPS.
Restoration and conservation efforts outlined in site-specific plans are guided by the Big Hole SHCP, a science-based framework for making management decisions and prioritizing where and how to deliver conservation efficiently to achieve specific biological outcomes for Arctic grayling. The SHCP delineates four spatial “tiers” that help prioritize where conservation will most benefit Arctic grayling:
(1) Tier I is 84 miles of core spawning, rearing and adult habitat that is currently occupied by Arctic grayling;
(2) Tier II is 91 miles of periphery habitat intermittently used by Arctic grayling;
(3) Tier III is 161 miles of suitable, but currently unoccupied, historical habitat; and
(4) Tier IV is 33 miles of potentially suitable habitat with unknown historical occupancy.
For reference, lands currently enrolled in the CCAA include 86 percent of tier I, 73 percent of tier II, 42 percent of tier III, and 24 percent of tier IV habitats. Given that the conservation measures outlined in the CCAA directly address known threats to Arctic grayling and their habitat in the Big Hole River, and that all conservation actions are strategically prioritized through the SHCP, the Service is encouraged by the positive habitat and Arctic grayling response to the conservation actions in the Big Hole River.
Conservation Efforts by Landowners Not Enrolled in the CCAA
Since 2006, twelve landowners in the Big Hole Valley who are not enrolled in the Big Hole CCAA have implemented voluntary conservation measures to benefit Arctic grayling. These conservation measures are similar to the conservation measures outlined in the SSP’s of landowners enrolled in the CCAA, including irrigation withdrawal reductions, installation of fish passage ladders, riparian fencing, stream restoration, and installation of stockwater tanks (MFWP 2014f, unpublished data). In addition, several of these landowners have informal flow agreements where the landowners have agreed to not utilize water returned to the stream by upstream enrollees in the Big Hole CCAA (MFWP 2014f, unpublished data). Although the majority of conservation projects in the Big Hole are completed through the CCAA, the Service is very encouraged by the participation of non-enrolled landowners to further grayling conservation in the Big Hole River and its tributaries.
Big Hole River Drought Management Plan
The purpose of the Drought Management Plan (DMP) is to mitigate the effects of low stream flows and lethal water temperatures for fisheries (particularly fluvial Arctic grayling) through a voluntary effort among participants including agriculture, municipalities, business, conservation groups, anglers, and affected government agencies (Big Hole Watershed Committee 2014, p.1). The DMP outlines flow triggers that, when met, initiate specific voluntary actions to conserve water. The flow triggers in the DMP are the same as the flow targets outlined in the Big Hole CCAA. The DMP has been in effect since 1999.
One key difference between the DMP and the CCAA is that the DMP is in effect for the entire Big Hole River, not just the upper Big Hole River like the CCAA. Arctic grayling occur outside of the CCAA Management Area; thus, any conservation efforts occurring in these areas still likely benefit Arctic grayling, although Arctic grayling densities outside the CCAA Management Area are low and represent a small fraction of the total population inside the CCAA Management Area (MFWP 2013d, unpublished data). Another key difference is that the DMP is structured to disseminate flow and water temperature information to all users of the Big Hole River, not just private landowners in the CCAA. This structuring allows for near real-time information sharing that helps inform users when voluntary conservation actions are needed. Such actions include reductions in irrigation withdrawal (for downstream users not in the CCAA); reductions in municipal, industrial, and personal water use; and reductions in recreation (e.g., angling).
The extent and magnitude of beneficial effects to Arctic grayling from the voluntary conservation measures recommended in the DMP are unclear. However, the DMP appears to have broad-based support. Most participants reduce irrigation withdrawals in response to observed low flows on nearby USGS gauges, before phone calls are made to request irrigation reductions (Downing 2014, pers. comm.). Increases in instream flow attributable to efforts under the DMP have been observed as “bumps” in the hydrograph in the middle and lower reaches of the Big Hole River (Downing 2014, pers. comm.). Although difficult to quantify, these “bumps” typically result in instream flows rising above low flow triggers (Downing 2014, pers. comm.). In addition, the inherent value of information sharing among diverse stakeholder groups about the potential effects of dewatering and thermal stress on the Big Hole fishery is likely significant. An increased understanding of conservation efforts needed to benefit Arctic grayling, and aquatic habitat in general, has been demonstrated to be a necessary precursor for more formalized conservation actions, such as the creation and implementation of the Big Hole CCAA.
Native Arctic Grayling Genetic Reserves and Translocation
Given concern over the status of native Arctic grayling, the Montana Arctic Grayling Recovery Program (AGRP) was formed in 1987, to address conservation concerns for primarily the fluvial ecotype inhabiting the Big Hole River, and to a lesser extent the native adfluvial population in the Centennial Valley (Memorandum of Understanding (MOU) 2007, p. 2). The Arctic Grayling Workgroup (AGW) was established as an ad hoc technical workgroup of the AGRP. In 1995, the AGW finalized a restoration plan that outlined an agenda of restoration tasks and research, including management actions to secure the Big Hole River population, brood stock development, and a program to re-establish four additional fluvial populations (Montana AGW 1995, pp. 7-17).
Consequently, Montana Fish, Wildlife and Parks established genetic reserves of Big Hole River and Centennial Valley Arctic grayling (Leary 1991, entire). Currently, brood (genetic) reserves of Big Hole River Arctic grayling are held in two closed-basin lakes in south-central Montana (Rens and Magee 2007, p. 22). These fish are manually spawned to provide gametes for translocation efforts in Montana (e.g., Ruby River population) (Rens and Magee 2007, p. 22). A brood reserve of Centennial Valley Arctic grayling has recently been established in Elk Lake; however, no natural reproduction has been documented since the brood reserve was established. Instream flows in the sole spawning tributary (Narrows Creek) to Elk Lake have been low in recent years, likely as a result of low snowpack in some years and seismic activity that altered the hydrology of Narrows Creek (Jaeger 2014c, pers. comm.). Future conservation actions on Narrows Creek include securing a more consistent water supply during the Arctic grayling spawning season through a water rights exchange; however, at this time, these conservation actions and the future viability of the Elk Lake population are too uncertain to warrant consideration in this finding.
A reintroduction effort in the upper Ruby River, where Arctic grayling were previously extirpated, using Big Hole River genetic reserves recently concluded. Arctic grayling eggs from the Big Hole River reserves were hatched on-site in incubators, and fry were allowed to drift into the reintroduction area. Supplementation of the Ruby River population concluded in 2008. For the last 5 years since then, natural reproduction has been documented in the upper Ruby River (Cayer and McCullough 2013, p. 21). Recent genetic analyses of the Ruby River population indicate high levels of genetic heterozygosity and allelic richness, albeit low estimate of effective number of breeders (Leary 2014, unpublished data). It has been hypothesized that the population is likely still expanding. Encouragingly, the number of breeding adults has trended upward over the past 3 years (Leary 2014, unpublished data). Most experts participating in the SSA workshop indicated that the Ruby River population was viable, given the evidence of natural reproduction occurring over the last 5 years at rates sufficient to increase the number of breeding adults over the past 3 years. Thus, we conclude the Ruby River population is viable.
Another recent conservation effort using Big Hole River genetic reserves involves an assisted recolonization effort of Arctic grayling in Rock Creek, a historically occupied tributary of the upper Big Hole River. Since 2010, incubators placed directly on location have been used to reintroduce young-of-year Arctic grayling into Rock Creek. Recolonization efforts are scheduled to be implemented in Rock Creek through 2015. Encouragingly, young-of-year and older Arctic grayling have been documented in 4 miles of Rock Creek over the past several years. This increase of Arctic grayling abundance and distribution in Rock Creek is likely due, at least in part, to the introduction of thousands of fry via the onsite incubators. Habitat improvement projects on Rock Creek have occurred simultaneously with fry reintroduction, so it is difficult to distinguish the relative effects of fry reintroduction and habitat improvement on the resulting increase in distribution and abundance of young Arctic grayling. Likely, both factors have played a role in reestablishing Arctic grayling in Rock Creek. Regardless, both conservation actions are having their intended effect: Increasing Arctic grayling abundance and distribution in historically occupied habitat.
In 2013, an increase in the number of breeding Arctic grayling was observed in the Big Hole River (Leary 2014, unpublished data). Given that fry were being reintroduced into the Big Hole River (and Rock Creek) beginning in 2010, there was initial uncertainty about the relative contribution of RSI-produced fish to the observed increase in breeding adults. Genetic analysis of a sample of young Arctic grayling obtained in 2013 indicated a low level of relatedness (<10 percent of sample were half- or full siblings) among individuals within the sample (Leary 2014, unpublished data). These results indicate that RSI-produced fish in 2010 contributed very little to the increase in breeding adults in 2013, as we would have expected a high degree of relatedness within the 2013 sample due to a small number of grayling spawned to produce eggs for the RSI reintroduction effort. Thus, these data suggest that factors other than the influence of RSIs were responsible for increasing abundance of adult spawners in the Big Hole River in 2013.
Similar reintroductions to the Rock Creek effort are also underway in several other tributaries and lakes within the upper Big Hole drainage and elsewhere, including the Wise River, Trail Creek, Twin Lakes and the Madison River. This suite of reintroductions is scheduled to occur for 5 years and conclude in 2018. However, the effectiveness of these reintroductions has not yet been assessed.
In the Centennial Valley, RSIs have been used fairly extensively to try to establish spawning runs of adult Arctic grayling in multiple tributaries to Upper Red Rock Lake (Boltz and Kaeding 2002, entire; Jaeger 2014d, pers. comm.). Thus far, these attempts have failed, although it is possible that fry produced by these efforts spawned in Red Rock Creek, instead of returning to the stream in which they were hatched (Mogen 2014, pers. comm). Recently, RSIs were used in Red Rock Creek in 2010, as part of a mitigation strategy to offset the removal of thousands of Arctic grayling eggs being taken to develop a brood reserve in Elk Lake. Similar to the Rock Creek example in the Big Hole, there was initial uncertainty whether recent increases in spawner abundance of Arctic grayling in Red Rock Creek were a direct result of the introduction of thousands of fry directly into Red Rock Creek via RSIs. However, fry produced in 2010 would not have contributed to the increases in spawning adult Arctic grayling observed in Red Rock Creek in 2010. Additionally, lengths of all adult Arctic grayling handled during the 2012 spawning run showed minimal overlap (<5 percent) with the length range of 2-year-old Arctic grayling from Upper Red Rock Lake, indicating fry produced in 2010 had little potential to contribute to the observed increase in adult spawners in Red Rock Creek in 2012 (Patterson 2014, unpublished data). The effect of RSI-produced Arctic grayling fry on abundance of spawners in Red Rock Creek after 2012 is unknown. The Service hopes that using RSIs as a conservation tool will result in RSI-produced fish recruiting to the Centennial Valley population.
Another Arctic grayling reintroduction project is currently being planned in Grayling Creek within Yellowstone National Park. Approximately 30 miles of historically occupied habitat are proposed for the reintroduction. Recently, a fish barrier was installed at the downstream extent of this habitat, and removal of all fish currently above the barrier commenced. Another round of fish removal is scheduled for the summer of 2014, to ensure complete removal of all existing nonnative fishes. Arctic grayling are scheduled to be reintroduced as early as 2015. Although the Service is encouraged by the potential for the reintroduction in 30 miles of historical habitat in Grayling Creek, it is unclear at this time if funding will be available to complete the project. Thus, although we are aware of the future potential of this project to contribute to Arctic grayling conservation, we do not consider this project to contribute to Arctic grayling conservation currently, and have not considered it as part of this status review or our listing determination for this DPS.
Summary of Factor A
Based on the best available information, we find that the historical range of the Missouri River DPS of Arctic grayling has been reduced particularly by large-scale habitat fragmentation by dams. However, despite fragmentation, sufficient habitat remains intact and is currently supporting multiple, viable, fluvial and adfluvial Arctic grayling populations. Historical threats to habitat quantity and quality in the Big Hole River are systematically being eliminated or minimized by the CCAA and SHCP through conservation projects designed to expressly address the four conservation criteria outlined in the CCAA. Large-scale habitat improvements are occurring; quality of riparian areas has improved in both the Big Hole River and Centennial Valley through riparian restoration projects, and these projects are expected to minimize effects of climate change through blocking of some solar radiation and channel morphology changes. In addition, Arctic grayling populations are responding favorably to habitat improvements in both the Big Hole River and Centennial Valley. In the future, we do not expect habitat to decline in the Big Hole River because of the proven track record of CCAA projects. In the Centennial Valley, protections provided by the NWR have sufficiently minimized past threats to habitat. These protections are expected to persist into the future and maintain the integrity of the habitat. Most of the other adfluvial populations of Arctic grayling reside in high-quality habitats on Federal land where mechanisms exist to conserve that habitat. Thus, we have no evidence that past threats under Factor A are acting currently on the Upper Missouri River DPS of Arctic grayling at the population or DPS level and no expectation that those impacts will pose a threat to the DPS in the future.
B. Overutilization for Commercial, Recreational, Scientific, or Educational Purposes
Arctic grayling of the upper Missouri River are handled for recreational angling and for scientific, population monitoring, and restoration purposes.
Recreational Angling
Arctic grayling are highly susceptible to capture by angling (ASRD 2005, pp. 19-20), and intense angling pressure can reduce densities and influence the demography of exploited populations (Northcote 1995, pp. 171-172). Historically, overfishing likely contributed to the rangewide decline of the DPS in the upper Missouri River system (Vincent 1962, pp. 49-52, 55; Kaya 1992, pp. 54-55). In 1994, concern over the effects of angling on fluvial Arctic grayling led the State of Montana to implement catch-and-release regulations for Arctic grayling captured in streams and rivers within its native range, and those regulations remain in effect today (MFWP 2014d, p. 51). Catch-and-release regulations also are in effect for Ennis Reservoir on the Madison River (MFWP 2014d, p. 59). Angling is not permitted in either of the Red Rock Lakes in the Centennial Valley to protect breeding waterfowl and trumpeter swans (Cygnus buccinator) (USFWS 2009, p. 147), and catch-and-release regulations remain in effect for any Arctic grayling captured in streams (e.g., Odell Creek or Red Rock Creek) in the Centennial Valley (MFWP 2014d, p. 67). Additionally, angling is closed in Red Rock Creek during the Arctic grayling spawning period (May 15 to June 14; MFWP 2014d, p. 67). However, we do note that Red Rock Creek is open to angling for other species (e.g., hybrid cutthroat trout) the remainder of the year and that Arctic grayling are caught during this time, particularly before the May 15 closure.
In all other populations, anglers can keep up to 5 Arctic grayling per day and have up to 10 in possession, in accordance with standard daily and possession limits for that angling management district (MFWP 2014d, p. 51). The population trends of Arctic grayling in many of the lakes (see Table 3, above) suggest that present angling exploitation rates are not a threat to those populations, even though harvest is allowed on most of these populations. Limited data preclude population estimates and trend inferences for some adfluvial populations (see Table 3).
Repeated catch-and-release angling may harm individual fish, causing physiological stress and injury (i.e., hooking wounds). Catch-and-release angling also can result in mortality at a rate dependent on hooking location, hooking duration, fish size, water quality, and water temperature (Faragher et al. 2004, entire; Bartholomew and Bohnsack 2005, p. 140; Boyd et al. 2010). Repeated hooking (up to five times) of Arctic grayling in Alaska did not result in significant additional mortality (rates 0 to 1.4 percent; Clark 1991, pp. 1, 25-26). In Michigan, hooking mortality of Arctic grayling in lakes averaged 1.7 percent per capture event, based on 355 individuals captured with artificial flies and lures (Nuhfer 1992, pp. 11, 29). Higher mortality rates (5 percent) have been reported for Arctic grayling populations in the Great Slave Lake area, Canada (Falk and Gillman 1975, cited in Casselman 2005, p. 23). Comparatively high catch rates for Arctic grayling have been observed in the Big Hole River, Montana (Byorth 1993, pp. 26-27, 36), and average hooking wound rates ranged from 15 to 30 percent among study sections (Byorth 1993, p. 28). However, overall hooking mortality from single capture events was low (1.4 percent), which led Byorth to conclude that the Big Hole River population was not limited by angling (Byorth 1994b, entire).
Compared to the average catch-and-release mortality rates of 4.2 to 4.5 percent in salmonids as reported by Schill and Scarpella (1997, p. 873), and the mean and median catch-and-release mortality rates of 18 percent and 11 percent from a meta-analysis of 274 studies (Bartholomew and Bohnsack 2005, pp. 136-137), the catch-and-release mortality rates for Arctic grayling are comparatively low (Clark 1991, pp. 1, 25-26; Nuhfer 1992, pp. 11, 29; Byorth 1994b, entire). We are uncertain whether these lower observed rates reflect an innate resistance to effects of catch-and-release angling in Arctic grayling or whether they reflect differences among particular populations or study designs used to estimate mortality. Even if catch-and-release angling mortality is low (e.g., 1.4 percent as reported in Byorth 1994b, entire), the high catchability of Arctic grayling (ASRD 2005, pp. 19-20) raises some concern about the cumulative mortality of repeated catch-and-release captures. For example, based on the Arctic grayling catch rates and angler pressure reported by Byorth (1993, pp. 25-26) and the population estimate for the Big Hole River reported in Byorth (1994a, p. ii), a simple calculation suggests that age 1 and older Arctic grayling susceptible to recreational angling may be captured and released 3 to 6 times per year.
In conclusion, angling harvest may have significantly reduced the abundance and distribution of the Upper Missouri River DPS of Arctic grayling during the past 50 to 100 years, but current catch-and-release fishing regulations (or angling closures) in most waters occupied by extant populations have likely ameliorated the past threat of overharvest. Although we do note the potential for cumulative mortality caused by repeated catch-and-release of individual Arctic grayling in the Big Hole River, we have no evidence indicating that repeated capture of Arctic grayling under catch-and-release regulations is currently limiting that population or the DPS. Moreover, fishing is restricted in the Big Hole River, an important recreational fishing destination in southwestern Montana, when streamflow and temperature conditions are likely to increase stress to captured Arctic grayling. Anglers can still capture and keep Arctic grayling in most lake populations in accordance with State fishing regulations, but we have no evidence that current levels of angling are affecting these populations. Thus, the best available evidence does not indicate that recreational angling represents a current threat to the DPS. We have no information at this time to indicate that future fishing regulations are likely to change in a way that would be detrimental to Arctic grayling. Thus, we do not believe that recreational angling will represent a threat in the future.
Monitoring and Scientific Study
Montana Fish, Wildlife and Parks consistently monitors the Arctic grayling population in the Big Hole River and its tributaries, and to a lesser extent those populations in the Madison River and Centennial Valley (Cayer and McCullough 2013, entire). Electrofishing (use of electrical current to temporarily and non-lethally immobilize a fish for capture) is a primary sampling method to monitor Arctic grayling in these populations (Rens and Magee 2007, pp. 13, 17, 20). A number of studies have investigated the effects of electrofishing on various life stages of Arctic grayling. Dwyer and White (1997, p. 174) found that electrofishing reduced the growth of juvenile Arctic grayling and concluded that long-term, sublethal effects of electrofishing were possible. Hughes (1998, pp. 1072, 1074-1075) found evidence that electrofishing and tagging affected the growth rate and movement behavior of Arctic grayling in the Chena River, Alaska. Roach (1999, p. 923) studied the effects of electrofishing on fertilized Arctic grayling eggs and found that while electrofishing could result in egg mortality, the population-level effects of such mortality were not likely to be significant. Lamothe and Magee (2003, pp. 16, 18-19) noted mortality of Arctic grayling in the Big Hole River during a radio-telemetry study, and concluded that handling stress or predation were possible causes of mortality. However, population monitoring activities in the Big Hole River are curtailed when environmental conditions become unsuitable (Big Hole Watershed Committee 1997, entire), and recent monitoring reports (Cayer and McCullough 2012, 2013, entire) provide no evidence that electrofishing is harming the Arctic grayling population in the Big Hole River.
Traps, electrofishing, and radio telemetry have been used to monitor and study Arctic grayling in the Centennial Valley (Gangloff 1996, pp. 13-14; Mogen 1996, pp. 10-13, 15; Kaeding and Boltz 1999, p. 4; Rens and Magee 2007, p. 17); however, there are no data to indicate these monitoring activities reduce the growth and survival of individual Arctic grayling or otherwise constitute a current or future threat to the population.
The Arctic grayling population in the Madison River-Ennis Reservoir is not monitored as intensively as the Big Hole River population (Rens and Magee 2007, pp. 20-21). When electrofishing surveys targeting Arctic grayling in the Madison River occur, they are conducted during the spawning run for that population (Clancey 1996, p. 6). Capture and handling during spawning migrations or during actual spawning could affect the reproductive success of individual Arctic grayling. However, under recent monitoring frequencies, any population-level effect of these activities is likely negligible, and we have no data to indicate these monitoring activities reduce the growth and survival of individual Arctic grayling or otherwise constitute a current or future threat to the Madison River population.
Most of the adfluvial populations of Arctic grayling are infrequently monitored (MFISH 2014a, unpublished data). Because monitoring of these populations has been minimal, we do not believe that monitoring or scientific study constitutes a current or future threat to these particular populations.
The intensity of monitoring and scientific investigation varies among the different populations in the DPS, but we have no evidence suggesting that monitoring or scientific study has influenced the decline of Arctic grayling in the Missouri River basin. We also have no evidence indicating these activities constitute a current threat to the DPS that would result in measurable, population-level effects. We expect similar levels of population monitoring and scientific study in the future, and we conclude that these activities will not represent a threat in the future.
Reintroduction Efforts
Attempts to restore or re-establish native populations of both fluvial and adfluvial Arctic grayling may result in the mortality of some embryos and young fish. Currently, gametes (eggs and sperm) used to re-establish the fluvial ecotype come from captive brood reserves of Big Hole River Arctic grayling maintained in Axolotl and Green Hollow II Lakes (Rens and Magee 2007, pp. 22-24). Removal of gametes from the wild Big Hole River population was necessary to establish this brood reserve (Leary 1991, entire) and will likely continue intermittently in the future to ensure the genetic representation of the brood reserve. The previous removal of gametes for conservation purposes could have hypothetically reduced temporarily the abundance of the wild population if the population was unable to compensate for this effective mortality by increased survival of remaining individuals. However, the establishment of a brood reserve provides a conservation benefit from the standpoint that gametes from the reserve can be harvested to use for translocation efforts to benefit the species. Ultimately, we conclude that past gamete collection from the Big Hole River population has not harmed the wild population or that collection in the future will harm the population. Consequently, we conclude that gamete collection from the Big Hole River Arctic grayling population does not constitute a current or future threat to the population.
Efforts to re-establish native, genetically representative populations of adfluvial Arctic grayling in the Centennial Valley and to maintain a brood reserve for that population have resulted in the direct collection of eggs from Arctic grayling spawning runs in Red Rock Creek. During 2000-2002, an estimated 315,000 Arctic grayling eggs were collected from females captured in Red Rock Creek (Boltz and Kaeding 2002, pp. v, 8). The Service placed over 180,000 of these eggs in remote site incubators in streams within the Red Rock Lakes NWR that historically supported Arctic grayling spawning runs (Boltz and Kaeding 2002, pp. v, 10).
Montana Fish, Wildlife and Parks and the Service are currently collaborating on an effort to re-establish an Arctic grayling spawning run in Elk Springs Creek and a replicate of the Centennial population in Elk Lake (West 2014a, pers. comm., Jaeger 2014e, pers. comm.). These actions required the collection of gametes (approximately 370,000 eggs) from Arctic grayling captured in Red Rock Creek (Jaeger 2014f, pers. comm.). Approximately 10 percent of these eggs were returned to Red Rock Creek and incubated in that stream (using a method resulting in high survivorship of embryos) (Kaeding and Boltz 2004, entire) to mitigate for collection of gametes from the wild spawning population. We infer that past gamete collection in Red Rock Creek has not significantly influenced recruitment in Red Rock Creek, as abundance of returning spawners to Red Rock Creek was robust in 2013 and 2014.
Overall, we conclude that collection of gametes from the wild populations in the Big Hole River and Centennial Valley systems has not contributed to population-level declines in those populations, or that the previous collections represent overexploitation. Future plans to collect gametes from Arctic grayling in the Big Hole River should be evaluated in light of the status of those populations at the anticipated time of the collections. We encourage the agencies involved to coordinate their efforts and develop a strategy for broodstock development and conservation efforts that minimizes any potential impacts to wild native populations. However, at present, we do not have any data indicating collection of gametes for conservation purposes represents a current threat to the Big Hole River and Centennial Valley populations. We have no evidence to indicate that gamete collection will increase in the future, so we conclude that this does not represent a future threat.
Conservation Efforts To Reduce Recreational Overutilization
The MFWP closes recreational angling in specific reaches of the Big Hole River when environmental conditions are considered stressful. Specific streamflow and temperature thresholds initiate mandatory closure of the fishery (Big Hole Watershed Committee 1997, entire). Such closures have been implemented as recently as 2013; however, changes to closure types and criteria in past years preclude any meaningful comparisons between different time periods (Horton 2014b, pers. comm.).
Summary of Factor B
Based on the best information available, we conclude that overexploitation by angling may have contributed to the historical decline of the Upper Missouri River DPS of Arctic grayling, but we have no evidence to indicate that current or future levels of recreational angling, population monitoring, scientific study, or conservation actions constitute overexploitation; therefore, we do not consider them a threat. We expect similar or decreased levels of these activities to continue in the future, and we do not believe they are likely to become a threat in the future.
Factor C. Disease or Predation
Disease
Arctic grayling are resistant to whirling disease, which is responsible for population-level declines of other stream salmonids (Hedrick et al. 1999, pp. 330, 333). However, Arctic grayling are susceptible to bacterial kidney disease (BKD), a bacterial disease causing reduced immune response and mortality in some fish species (Meyers et al. 1993, p. 181). Some wild populations in pristine habitats test positive for BKD (Meyers et al. 1993, pp. 186-187), but clinical effects of the disease are more likely to be evident in captive populations (Meyers et al. 1993, entire; Peterson 1997, entire). To preclude transmission of BKD between Arctic grayling during brood reserve, hatchery, and wild Arctic grayling translocation efforts, MFWP tests kidney tissue and ovarian fluid for the causative agent for BKD as well as other pathogens in brood populations (Rens and Magee 2007, pp. 22-24).
Information on the prevalence of BKD or other diseases in native Arctic grayling populations in Montana is generally lacking. One reason for this lack of information is that some disease assays are invasive or require the sacrifice of individual fish (e.g., removal of kidney tissue to test for BKD pathogen), so they are not done often on native populations. Arctic grayling in captive brood reserves (e.g., Axolotl Lake, Green Hollow Lake) have all recently tested negative for infectious hematopoietic necrosis virus (IHNV), infectious pancreatic necrosis virus (IPNV), Myxobolus cerebralis (the pathogen that causes whirling disease), Renibacterium salmoninarum (the pathogen that causes BKD), and Aeromonas salmonicida (the pathogen that causes furunculosis) (USFWS 2010b). Consequently, the best available evidence at this time does not indicate that disease threatens native Arctic grayling of the upper Missouri River. We have no basis to conclude that disease will become a future threat, so we conclude that disease does not constitute a threat in the future.
Predation By and Competition With Nonnative Trout
Brook trout (Salvelinus fontinalis), brown trout (Salmo trutta), and rainbow trout are widely distributed and abundant in the western United States, including the upper Missouri River system (Schade and Bonar 2005, p. 1386; Table 6). One or more of these nonnative trout species co-occur with 11 of the 20 Arctic grayling population in the basin. The remaining nine Arctic grayling populations occur with other native species or no other fish species (Table 6).
Ecological interactions (predation and competition) with the brook trout, brown trout, and rainbow trout are among the long-standing hypotheses to explain the historical decline of Arctic grayling in the upper Missouri River system and the extirpation of some populations from specific waters (Nelson 1954, p. 327; Vincent 1962, pp. 81-96; Kaya 1992, pp. 55-56). Strength of competition and predation can be very difficult to measure in wild trout populations (Fausch 1988, pp. 2238, 2243; 1998, pp. 220, 227). Predation on Arctic grayling eggs and fry by brook trout has been observed in both the Big Hole River and the Centennial Valley (Nelson 1954, entire; Streu 1990, p. 17; Katzman 1998, pp. 35, 47, 114), but such observations have not been definitively linked to population declines of Arctic grayling. To our knowledge, no studies have investigated or attempted to measure predation by brown trout or rainbow trout on Arctic grayling in Montana. Brook trout do not appear to negatively affect habitat use or growth of juvenile, hatchery-reared Arctic grayling (Byorth and Magee 1998, p. 921), but further studies are necessary to determine whether competition or predation occur at other life stages or with brown or rainbow trout (Byorth and Magee 1998, p. 929). Predation represents direct mortality that can limit populations, and young-of-year Arctic grayling may be particularly susceptible to predation by other fishes because they are smaller and weaker swimmers than trout fry (Kaya 1990, pp. 52-53).
The evidence for predation and competition by nonnative trout on Arctic grayling in the upper Missouri River basin is largely circumstantial, and inferred from the reduced historical abundance and distribution of Arctic grayling following encroachment by nonnative trout (Kaya 1990, pp. 52-54; Kaya 1992, p. 56; Magee and Byorth 1995, p. 54). In addition, the historical difficulty in establishing Arctic grayling populations in waters already occupied by nonnative trout, especially brown trout (Kaya 2000, pp. 14-15) may suggest competition and predation play a role. However, the often-cited case histories where nonnative trout were implicated in the decline of Arctic grayling also involved prior or concurrent habitat modification or degradation, thus confounding the two factors (Kaya 1990, pp. 52-54; Kaya 1992, p. 56; Magee and Byorth 1995, p. 54) and making it difficult to pinpoint the cause of the decline. Where past habitat degradation has not been a factor (e.g., many of the high-elevation adfluvial populations), successful coexistence between brook trout and rainbow trout and Arctic grayling has occurred over long durations, greater than 100 years in some populations (Jaeger 2014, unpublished data; MFISH 2014a, unpublished data). Despite past habitat degradation in the Big Hole River, Arctic grayling have coexisted with brook, rainbow and brown trout for at least 60 years (Liknes 1981, p. 34).
In the Big Hole River, brook trout, rainbow trout, and brown trout are more abundant than Arctic grayling (Rens and Magee 2007, p. 42). In general, brook trout is the most abundant nonnative trout species in the Big Hole River upstream from Wisdom, Montana (Rens and Magee 2007, pp. 7, 42; Lamothe et al. 2007, pp. 35-38), whereas rainbow trout and brown trout are comparatively more abundant in the downstream reaches (Kaya 1992, p. 56; Oswald 2005, pp. 22-29; Lamothe et al. 2007, pp. 35-38; Rens and Magee 2007, p. 10). Recently, brown trout abundance has increased in the upper Big Hole River upstream of Wisdom (MFWP 2013e, unpublished data). In the reach of the upper Big Hole River where Arctic grayling densities are highest, nonnative trout abundances are lower than upstream or downstream reaches, and appear to have been stable since at least 2006 (Cayer 2013, unpublished data).
The potential effects of nonnative trout species (rainbow, brown, brook, and Yellowstone cutthroat trout) on Arctic grayling recruitment are largely unknown. Arctic grayling experts from Montana convened to explore such effects predicted a less than 12 percent reduction in Arctic grayling recruitment when nonnative trout densities for any species were 500 fish/mile or fewer, on average (SSA 2014, p. 2). Predicted reduction in Arctic grayling recruitment when any of the nonnative species were present at 1,000 fish/mile was higher and similar among species (20 to 25 percent; SSA 2014, p. 2). These estimates were derived with the assumption that habitat was not a limiting factor.
Currently, densities of nonnative trout (brook, brown, rainbow) are fewer than 20 fish/mile (per species) in the mainstem Big Hole River where Arctic grayling densities are highest (Cayer 2013, unpublished data). Densities of brown and rainbow trout are fewer than 20 fish/mile in Big Hole River tributaries, while brook trout density in tributaries is higher (∼80 fish/mile). Brook trout density estimates only include fish greater than 10 inches, thus it is unknown how many total brook trout reside in these areas. At current densities of rainbow and brown trout, effects on Arctic grayling recruitment would be expected to be small, based on the predictions of recruitment reduction from nonnatives from the expert meeting.
In the Madison River in and near Ennis Reservoir, brown trout and rainbow trout are abundant and are the foundation of an important recreational fishery (e.g., Byorth and Shepard 1990, p. 1). Nonnative rainbow trout and brown trout densities in the Madison River near Ennis Reservoir are about 3,500 to 4,000 fish per mile (both species included). These densities are substantially higher than those observed in other systems occupied by Arctic grayling, and are higher than those asked of the Arctic grayling experts to predict effects of on Arctic grayling recruitment. Arctic grayling abundance in the Ennis Reservoir/Madison River population appears to be suppressed and declining (MFWP 2013f, unpublished data). The relationship between the higher densities of nonnative trout and the low and declining abundance of Arctic grayling in this population is unclear. However, the densities of nonnative trout observed in the Madison River are not representative of densities of nonnatives in any of the other 19 populations of Arctic grayling in the DPS. Thus, the effect of nonnatives on Arctic grayling recruitment is a concern in the Madison River, but not in the rest of the DPS.
In the Centennial Valley, brook trout and hybrid cutthroat trout (Yellowstone cutthroat trout (Oncorhynchus clarkii bouvieri) crossed with rainbow trout; Mogen 1996, p. 42) have well-established populations and dominate the abundance and biomass of the salmonid community (Katzman 1998, pp. 2-3; Boltz 2010, pers. comm.). In Upper Red Rock Lake, hybrid cutthroat trout and Arctic grayling exhibit some dietary overlap (Cutting 2012, unpublished data), although food may not be a limiting factor in this system, given the eutrophic, highly productive nature of Upper Red Rock Lake (Jaeger 2014g, pers. comm.). In addition, hybrid Yellowstone cutthroat trout in Upper Red Rock Lake may occupy a similar ecological niche once occupied by native westslope cutthroat trout, a species with which Arctic grayling co-evolved. Thus, the adaptations Arctic grayling developed over thousands of years to coexist with westslope cutthroat trout may be equally advantageous when coexisting with hybrid Yellowstone cutthroat trout.
Predation of Arctic grayling by brook trout and hybrid cutthroat trout occurs in Upper Red Rock Lake (Nelson 1954, entire; Katzman 1998, pp. 35, 47, 114). In 2013, the Service initiated a removal effort to suppress hybrid cutthroat trout in Red Rock Creek and Upper Red Rock Lake. This effort will occur for 5 years, during and after which the biological response of Arctic grayling will be documented. Currently, the relationship between hybrid cutthroat trout and Arctic grayling abundance in the Centennial Valley is unclear. However, a recent peak in hybrid cutthroat trout abundance was paralleled by a peak in Arctic grayling abundance, indicating predation by hybrid cutthroat trout is likely not a threat to Arctic grayling in the Centennial Valley. It is plausible that extensive macrophyte beds present in Upper Red Rock Lake (Katzman 1998, p. 81) provide complex hiding and rearing cover for juvenile Arctic grayling and minimize interactions between young Arctic grayling and nonnative fishes (Almany 2004, entire).
In the upper Missouri River basin, it appears that the extent and magnitude of competition and predation between nonnative trout and Arctic grayling likely depends on environmental context (e.g., habitat type and quality, environmental conditions such as temperature, etc.) in most populations. High-quality habitats likely provide more food resources and complexity (rearing areas) than lower quality habitats (MacArthur and Levins 1967, entire). These features of high-quality habitats probably lessen competition (MacArthur and Levins 1967, entire) and reduce predation (Almany 2004, p. 107) by providing complex rearing areas for the vulnerable young life stages of Arctic grayling. For these reasons historically, when many of the fluvial habitats were degraded, competition and predation likely had a larger effect of Arctic grayling populations than they currently do. Certainly, competition and predation are still occurring in habitats occupied by both nonnatives and Arctic grayling. However, the increase in habitat quality observed in recent years, particularly in the Big Hole River and Centennial Valley, appear to have minimized effects of competition and predation on respective Arctic grayling populations. The primary evidence of this is recent trends showing increasing numbers of both nonnatives and Arctic grayling in systems with high-quality habitat, including increasing brown trout and Arctic grayling in the Big Hole River. Other adfluvial populations of Arctic grayling have coexisted with brook, rainbow, and Yellowstone cutthroat trout for extended periods of time (>60 years) with no observed declines in abundance.
Predation by Birds and Mammals
In general, the incidence and effect of predation by birds and mammals on Arctic grayling is not well understood because few detailed studies have been completed (Northcote 1995, p. 163). Black bear (Ursus americanus), mink (Neovison vison), and river otter (Lontra canadensis) are present in southwestern Montana, but direct evidence of predatory activity by these species is often lacking (Kruse 1959, p. 348). Osprey (Pandion haliaetus) can capture Arctic grayling during the summer (Kruse 1959, p. 348). In the Big Hole River, Byorth and Magee (1998, p. 926) attributed the loss of Arctic grayling from artificial enclosures used in a competition experiment to predation by minks, belted kingfisher (Ceryle alcyon), osprey, and great blue heron (Ardea herodia). In addition, American white pelican (Pelecanus erythrorhynchos) are seasonally present in the Big Hole River, and they also may feed on Arctic grayling. The aforementioned mammals and birds can be effective fish predators; however, Arctic grayling evolved with these native predator species and have developed life-history and reproductive strategies to mitigate for predation losses. We have no data demonstrating any of these species historically or currently consume Arctic grayling at levels sufficient to exert a measureable, population-level impact on native Arctic grayling in the upper Missouri River system. We expect the current situation to continue, so we conclude that predation by birds and mammals does not constitute a threat to Missouri River Arctic grayling now or in the future.
Summary of Factor C
Based on the information available at this time, we conclude disease does not represent a past or current threat to the Upper Missouri River DPS of Arctic grayling. We have no basis for concluding that disease may become a future threat.
Predation and competition can influence the distribution, abundance, and diversity of species in ecological communities. Predation by and competition with nonnative species can negatively affect native species, particularly those that are stressed or occurring at low densities due to unfavorable environmental conditions. Historically, the impact of predation and competition from nonnatives was likely greater because many of the habitats used by Arctic grayling were degraded. Thus, predation and competition likely played a role historically in decreasing the abundance and distribution of Arctic grayling. Currently, habitat conditions have improved markedly for those Arctic grayling populations on Federal land (18 of 20 populations) and for the Big Hole River population on primarily private land. Predation and competition with nonnative species are still occurring in these systems, although the extent and magnitude of these effects appears to be mediated by habitat quality. Abundance of Arctic grayling and nonnative brown trout are increasing in the Big Hole River. Before suppression efforts began, Yellowstone cutthroat hybrids and Arctic grayling spawners were both at 40 year highs in Red Rock Creek in the Centennial Valley. We acknowledge nonnative trout densities are high in the Madison River and may be contributing to the decline of that Arctic grayling population; however, most other adfluvial populations appear to have stable abundance of Arctic grayling and nonnatives. Thus, based on our review we have no information that predation or competition represents a threat at the DPS level on the Upper Missouri River DPS of Arctic grayling. Further, Arctic grayling experts project only a small effect of predicted nonnative trout densities on Arctic grayling recruitment in the future. Thus, we have no information that predation or competition from nonnative trout represents a future threat at the population or species level.
Little is known about the effect of predation on Arctic grayling by birds and mammals. Such predation likely does occur, but we are not aware of any situation where an increase in fish-eating birds or mammals has coincided with the decline of Arctic grayling. Consequently, the available information does not support a conclusion that predation by birds or mammals represents a substantial past, present, or future threat to native Arctic grayling in the upper Missouri River.
Factor D. The Inadequacy of Existing Regulatory Mechanisms
Section 4(b)(1)(A) of the Act requires the Service to take into account “those efforts, if any, being made by any State or foreign nation, or any political subdivision of a State or foreign nation, to protect such species . . .” We consider relevant Federal, State, and Tribal laws, and regulations when evaluating the status of the species. Regulatory mechanisms, if they exist, may preclude the need for listing if we determine that such mechanisms adequately address the threats to the species such that listing is not warranted. Only existing ordinances, regulations, and laws, that have a direct connection to a law, are enforceable and permitted are discussed in this section. All other measures are discussed under the specific relevant factor.
U.S. Federal Laws and Regulations
No Federal laws in the United States specifically address the Arctic grayling, but several, in their implementation, may affect the species’ habitat.
National Environmental Policy Act
All Federal agencies are required to adhere to the National Environmental Policy Act (NEPA) of 1970 (42 U.S.C. 4321 et seq.) for projects they fund, authorize, or carry out. The Council on Environmental Quality’s regulations for implementing NEPA (40 CFR parts 1500-1518) state that, when preparing environmental impact statements, agencies shall include a discussion on the environmental impacts of the various project alternatives, any adverse environmental effects which cannot be avoided, and any irreversible or irretrievable commitments of resources involved (40 CFR part 1502). The NEPA itself is a disclosure law, and does not require subsequent minimization or mitigation measures by the Federal agency involved. Although Federal agencies may include conservation measures for Arctic grayling as a result of the NEPA process, any such measures are typically voluntary in nature and are not required by NEPA.
Federal Land Policy and Management Act
The Federal Land Policy and Management Act (FLPMA) of 1976 (43 U.S.C. 1701 et seq.), as amended, states that the public lands shall be managed in a manner that will protect the quality of scientific, scenic, historical, ecological, environmental, air and atmospheric, water resource, and archeological values. This statute protects lands within the range of the Arctic grayling managed by the Bureau of Land Management (BLM).
The BLM considers the fluvial Arctic grayling a sensitive species requiring special management consideration for planning and environmental analysis (BLM 2009a, entire, BLM 2009b, entire). The BLM has recently developed a resource management plan (RMP) for the Dillon Field Office Area that provides guidance for the management of over 900,000 acres of public land administered by BLM in southwest Montana (BLM 2006a, p. 2). The Dillon RMP area thus includes the geographic area that contains the Big Hole, Miner, Mussigbrod, Madison River, and Centennial Valley populations of Arctic grayling. A RMP planning area encompasses all private, State, and Federal lands within a designated geographic area (BLM 2006a, p. 2), but the actual implementation of the RMP focuses on lands administered by the BLM that typically represent only a fraction of the total land area within that planning area (BLM 2006b, entire). Restoring Arctic grayling habitat and ensuring the long-term persistence of both fluvial and adfluvial ecotypes are among the RMP’s goals (BLM 2006a, pp. 30-31). However, there is little actual overlap between the specific parcels of BLM land managed by the Dillon RMP and the current distribution of Arctic grayling (BLM 2006b, entire).
The BLM also has a RMP for the Butte Field Office Area, which includes more than 300,000 acres in south-central Montana (BLM 2008, entire), including portions of the Big Hole River in Deerlodge and Silver Bow counties (BLM 2008, p. 8; 2009c, entire). The Butte RMP considers conservation and management strategies and agreements for Arctic grayling in its planning process and includes a goal to opportunistically enhance or restore habitat for Arctic grayling (BLM 2008, pp. 10, 30, 36). However, the Butte RMP does not mandate specific actions to improve habitat for Arctic grayling in the Big Hole River and little overlap exists between BLM-managed lands and Arctic grayling occupancy in this planning area.
National Forest Management Act
Under the U.S. Forest Service (USFS) National Forest Management Act (NFMA) of 1976, as amended (16 U.S.C. 1600 et seq.), the USFS strives to provide for a diversity of plant and animal communities when managing national forest lands. Individual national forests may identify species of concern that are significant to each forest’s biodiversity. The USFS considers fluvial Arctic grayling a sensitive species (USFS 2004, entire) for which population viability is a concern. However, this designation provides no special regulatory protections.
Most of the upper Missouri River grayling populations occur on National Forest land; all 16 adfluvial populations and the fluvial Ruby River population (majority on National Forest) occur on USFS-managed lands. These populations occur across four different National Forests; consequently the riparian habitats surrounding the lakes and tributaries are managed according to the standards and guidelines outlined in each National Forest Plan. All Forest Plans do not contain the same standards and guidelines; however, each Plan has standards and guidelines for protecting riparian areas around perennial water sources. In the Beaverhead-Deerlodge and Helena National Forest Plans, the Inland Native Fish Strategy (INFS) standards and guidelines have been incorporated. The INFS, in part, defines widths of riparian buffer zones adequate to protect streams and lakes from non-channelized sediment inputs and contribute to other riparian functions, such as stream shading and bank stability. These protections have been incorporated into the Beaverhead-Deerlodge and Helena National Forest Plans through amendments and are currently preserving intact riparian areas around most, if not all, adfluvial Arctic grayling habitats. Exceptions to the riparian protections outlined in INFS are occasionally granted; however, these exceptions require an analysis of potential effects and review by a USFS fish biologist.
On the Gallatin National Forest, standards and guidelines in the Forest Plan include using “best management practices (BMPs)” to protect water sources and riparian areas. Similar to INFS, BMPs outline buffer strips along watercourses where disturbance and activity is minimized to protect riparian areas and water quality. On the Lewis and Clark National Forest, standards and guidelines are in place to leave timbered buffer strips adjacent to waterbodies to protect riparian areas. Grayling habitat on the Gallatin and Lewis and Clark National Forests consists of seven high-elevation mountain lakes.
The NFMA and INFS are adequately protecting riparian habitat on National Forest land, given the intact nature of most riparian areas surrounding the high-elevation lake populations and the Ruby River.
National Park Service (NPS) Organic Act
The NPS Organic Act of 1916 (16 U.S.C. 1 et seq.), as amended, states that the NPS “shall promote and regulate the use of the Federal areas known as national parks, monuments, and reservations . . . to conserve the scenery and the national and historic objects and the wild life therein and to provide for the enjoyment of the same in such manner and by such means as will leave them unimpaired for the enjoyment of future generations.” Arctic grayling are native to the western part of Yellowstone National Park and habitats are managed accordingly for the species under the Native Species Management Plan (NPS 2010, entire). One adfluvial Arctic grayling population, Grebe Lake, currently occurs in Yellowstone National Park. The Grebe Lake population is one of the larger adfluvial populations (see Table 3, above) in the DPS. The habitat in Grebe Lake and the tributaries is managed for conservation (NPS 2010, p. 44). Further, it is expected that these habitats will be managed for conservation in the future, based on provisions in the Organic Act and guidance outlined in the Native Species Management Plan.
National Wildlife Refuge System Improvement Act of 1997
The National Wildlife Refuge Systems Improvement Act (NWRSIA) of 1997 (Pub. L. 105-57) amends the National Wildlife Refuge System Administration Act of 1966 (16 U.S.C. 668dd et seq.). The NWRSIA directs the Service to manage the Refuge System’s lands and waters for conservation. The NWRSIA also requires monitoring of the status and trends of refuge fish, wildlife, and plants. The NWRSIA requires development of a comprehensive conservation plan (CCP) for each refuge and management of each refuge consistent with its plan.
The Service has developed a final CCP to provide a foundation for the management and use of Red Rock Lakes NWR (USFWS 2009, entire) in the Centennial Valley. Since the development of the CCP, Refuge staff have conducted numerous habitat conservation/restoration projects to benefit Arctic grayling, including: Removal of an earthen dam whose reservoir inundated several hundred meters of historical Arctic grayling spawning habitat in Elk Springs Creek, and subsequent reintroductions and tracking of young-of-year Arctic grayling in Elk Springs Creek (West 2014a, pers. comm.). However to date, the reintroductions in Elk Springs Creek have not established a spawning run. Other conservation projects conducted on the Refuge include the acquisition of new land and decreases in grazing intensities from 20,000 AUMs to about 5,000 AUMs. The Refuge has implemented a rest-rotation grazing system where more durable lands are grazed while more sensitive lands (e.g., riparian areas) are rested for up to 4 years (West 2014a, pers. comm.). Some active riparian restoration has also occurred, including a project to reconnect Red Rock Creek to a historical channel and replacement of four culverts to allow for natural tributary migration across alluvial fans (West 2014a, pers. comm.). The Refuge is also actively engaged in supporting ongoing graduate research efforts to explore potential limiting factors for Arctic grayling in the Centennial Valley.
Other conservation projects under the CCP have been focused on potential nonnative species effects on Arctic grayling, namely a 5-year project removing hybrid cutthroat trout captured during their upstream spawning run and a study of dietary overlap between Arctic grayling and Yellowstone cutthroat trout (West 2014a, pers. comm.). The Refuge also operates a sill dam (previous upstream fish barrier) to provide upstream fish passage and operates one irrigation ditch only when snowpack is average or above and timing is such that young Arctic grayling are not present near the diversion (West 2014a, pers. comm.).
The NWRSIA is adequately protecting habitat for Arctic grayling on the Refuge because riparian habitats are improving and the Centennial Valley population is increasing in both abundance and distribution. The proven track record of completed conservation projects on the refuge and currently expanding Arctic grayling population indicate that the continued implementation of the CCP during the next 15 years (which is the life of the CCP) will continue to improve habitat conditions on the refuge.
Federal Power Act (FPA)
The Federal Power Act of 1920 (16 U.S.C. 791
et seq
., as amended) provides the legal authority for the Federal Energy Regulatory Commission (FERC), as an independent agency, to regulate hydropower projects. In deciding whether to issue a license, FERC is required to give equal consideration to mitigation of damage to, and enhancement of, fish and wildlife (16 U.S.C. 797(e)). A number of FERC-licensed dams exist in the Missouri River basin in current (i.e., Ennis Dam on the Madison River) and historical Arctic grayling habitat (e.g., Hebgen Dam on the Madison River; Hauser, Holter, and Toston dams on the mainstem Missouri River; and Clark Canyon Dam on the Beaverhead River). The FERC license expiration dates for these dams range from 2024 (Toston) to 2059 (Clark Canyon) (FERC 2010, entire). None of these structures provides upstream passage of fish, and such dams are believed to be one of the primary factors that led to the historical decline of Arctic grayling in the Missouri River basin (see discussion under Factor A, above). However, recent monitoring data indicate multiple stable Arctic grayling populations occurring above mainstem dams, with the exception of the Ennis Reservoir/Madison River population. The drawdowns in reservoir water level believed to have historically affected the Ennis Reservoir/Madison River Arctic grayling population are not permitted under a new licensing agreement between the Federal Energy Regulatory Commission and Madison Dam operators, as we described previously in this finding (Clancey 2014, pers. comm.). This change in water management in Ennis Reservoir will ensure adequate rearing and foraging habitat for this population. The fluvial ecotype is still represented in the DPS and both strictly fluvial Arctic grayling populations appear to be stable or increasing. Thus, we conclude the Federal Power Act is currently adequate to protect the Upper Missouri River DPS of Arctic grayling at the population and DPS level.
Clean Water Act
The Clean Water Act (CWA) of 1972 (33 U.S.C. 1251 et seq.) establishes the basic structure for regulating discharges of pollutants into the waters of the United States and regulating quality standards for surface waters. The CWA’s general goal is to “restore and maintain the chemical, physical, and biological integrity of the Nation’s waters” (33 U.S.C. 1251(a)). The CWA requires States to adopt standards for the protection of surface water quality and establishment of total maximum daily load (TMDL) guidelines for rivers. The Big Hole River has approved TMDL plans for its various reaches (MDEQ 2009a, entire; 2009b, entire); thus, complete implementation of this plan should improve water quality (by reducing water temperatures, and reducing sediment and nutrient inputs) in the Big Hole River in the future. As of September 2013, there was no significant TMDL plan development activity in the Madison River or Red Rock watershed in the Centennial Valley (see MDEQ 2014). Currently, TMDL documents have been approved for the Ruby River. All planning areas containing other adfluvial Arctic grayling populations in the upper Missouri River basin have approved TMDLs, including the Gallatin, Lake Helena, and Sun watersheds (see MDEQ 2014).
Currently, water temperatures in the Big Hole River exceed levels outlined in the TMDL. However, reductions in water temperature within tributaries have been demonstrated (see discussion under Factor A and Table 4). Given that most Arctic grayling populations within the upper Missouri River basin are stable or increasing and habitats are largely being managed in a manner that benefits the species, we have no evidence that the CWA is inadequately protecting Arctic grayling at the population or DPS level.
State Laws
Montana Environmental Policy Act
The legislature of Montana enacted the Montana Environmental Policy Act (MEPA) as a policy statement to encourage productive and enjoyable harmony between humans and their environment, to protect the right to use and enjoy private property free of undue government regulation, to promote efforts that will prevent or eliminate damage to the environment and biosphere and stimulate the health and welfare of humans, to enrich the understanding of the ecological systems and natural resources important to the State, and to establish an environmental quality council (MCA 75-1-102). Part 1 of the MEPA establishes and declares Montana’s environmental policy. Part 1 has no legal requirements, but the policy and purpose provide guidance in interpreting and applying statutes. Part 2 requires State agencies to carry out the policies in Part 1 through the use of systematic, interdisciplinary analysis of State actions that have an impact on the human environment. This is accomplished through the use of a deliberative, written environmental review. In practice, MEPA provides a basis for the adequate review of State actions in order to ensure that environmental concerns are fully considered (MCA 75-1-102). Similar to NEPA, the MEPA is largely a disclosure law and a decision-making tool that does not specifically require subsequent minimization or mitigation measures.
Laws Affecting Physical Aquatic Habitats
A number of Montana State laws have a permitting process applicable to projects that may affect stream beds, river banks, or floodplains. These include the Montana Stream Protection Act (SPA), the Streamside Management Zone Law (SMZL), and the Montana Natural Streambed and Land Preservation Act (Montana Department of Natural Resources (MDNRC) 2001, pp. 7.1-7.2). The SPA requires that a permit be obtained for any project that may affect the natural and existing shape and form of any stream or its banks or tributaries (MDNRC 2001, p. 7.1). The Montana Natural Streambed and Land Preservation Act (i.e., MNSLPA or 310 permit) requires private, nongovernmental entities to obtain a permit for any activity that physically alters or modifies the bed or banks of a perennially flowing stream (MDNRC 2001, p. 7.1). The SPA and MNSLPA laws do not mandate any special recognition for species of concern, but in practice, biologists that review projects permitted under these laws usually stipulate restrictions to avoid harming such species (Horton 2010, pers. comm.). The SMZL regulates forest practices near streams (MDNRC 2001, p. 7.2). The Montana Pollutant Discharge Elimination System (MPDES) Stormwater Permit applies to all discharges to surface water or groundwater, including those related to construction, dewatering, suction dredges, and placer mining, as well as to construction that will disturb more than 1 acre within 100 ft (30.5 m) of streams, rivers, or lakes (MDNRC 2001, p. 7.2).
Review of applications by MFWP, MTDEQ, or MDNRC is required prior to issuance of permits under the above regulatory mechanisms (MDNRC 2001, pp. 7.1-7.2). These regulatory mechanisms are expected to limit impacts to aquatic habitats in general. Given that most Arctic grayling populations are stable or increasing in abundance in the presence of these regulatory mechanisms, we have no basis for concluding that these regulatory mechanisms are inadequate to protect the Arctic grayling and their habitat now or in the future.
Montana Water Use Act
The purpose of the Montana Water Use Act (Title 85: Chapter 2, Montana Codes Annotated) is to provide water for existing and future beneficial use and to maintain minimum flows and water quality in Montana’s streams. The Missouri River system is generally believed to be overappropriated, and water for additional consumptive uses is only available for a few months during very wet years (MDNRC 1997, p. 12). However, the upper Missouri River basin and Madison River basin have been closed to new water appropriations because of water availability problems, overappropriation, and a concern for protecting existing water rights (MDNRC 2009, p. 45). In addition, recent compacts (a legal agreement between Montana, a Federal agency, or an Indian tribe determining the quantification of federally or tribally claimed water rights) have been signed that close appropriations in specific waters in or adjacent to Arctic grayling habitats. For example, the USFWS-Red Rock Lakes-Montana Compact includes a closure of appropriations for consumptive use in the drainage basins upstream of the most downstream point on the Red Rock Lakes NWR and the Red Rock Lakes Wilderness Area (MDNRC 2009, pp. 18, 47). The NPS-Montana Compact specifies that certain waters will be closed to new appropriations when the total appropriations reach a specified level, and it applies to Big Hole National Battlefield and adjacent waters (North Fork of the Big Hole River and its tributaries including Ruby and Trail Creeks), and the portion of Yellowstone National Park that is in Montana (MDNRC 2009, p. 48).
The State of Montana is currently engaged in a Statewide effort to adjudicate (finalize) water rights claimed before July 1, 1973. The final product of adjudication in a river basin is a final decree. To reach completion, a decree progresses through several stages: (1) Examination, (2) temporary preliminary decree, (3) preliminary decree, (4) public notice, (5) hearings, and (6) final decree (MDNRC 2009, pp. 9-14). As of February 2014, the Centennial Valley has a preliminary decree, and the Big Hole and Madison Rivers have preliminary temporary decrees (MDNRC 2014, entire). We anticipate the final adjudication of all the river basins in Montana that currently contain native Arctic grayling will be completed in the next 5 years, but we do not know if this process will eliminate the overallocation of water rights. We note that the overallocation of water in some systems within the upper Missouri river basin is of general concern to Arctic grayling because of the species’ need for adequate quantity and quality of water for all life stages. However, we have no information indicating that overallocation of water in the upper Missouri River basin is a current threat at the individual or DPS level because most populations are stable or increasing at this time. Therefore, we conclude that the Montana Water Use Act is adequate to protect the Arctic grayling and its habitat.
Angling Regulations
Arctic grayling is considered a game fish (MFWP 2010, p. 16), but is subject to special catch-and-release regulations in streams and rivers within its native range, as was described under Factor B, above (MFWP 2014d, p. 51). Catch-and-release regulations also are in effect for Ennis Reservoir on the Madison River and Red Rock Creek in the Centennial Valley (MFWP 2014d, p. 63). Arctic grayling in other adfluvial populations are subject to more liberal regulations; anglers can keep up to 5 per day and have up to 10 in possession in accordance with standard daily and possession limits for that angling management district (MFWP 2014d, p. 51). We have no evidence to indicate that current fishing regulations are inadequate to protect native Arctic grayling in the Missouri River basin (see discussion under Factor B, above).
Summary of Factor D
Current Federal and State regulatory mechanisms are adequate to protect Arctic grayling of the upper Missouri River. We conclude this because the majority of populations are on Federal land where regulatory mechanisms are in place to preserve intact habitats and are expected to remain in place. In the Big Hole River, fluvial Arctic grayling generally occupy waters adjacent to private lands (MFWP et al. 2006, p. 13; Lamothe et al. 2007, p. 4), so Federal regulations may have limited ability to protect that population. However, some Federal regulations (e.g., CWA, FPA, NMFA, NWRSIA, NPS Organic Act) in concert with other existing conservation efforts (e.g., Big Hole CCAA) are adequate to sustain and improve habitat conditions for Arctic grayling. Arctic grayling in the Big Hole River appear to be responding positively to these improvements. In addition, we did not identify other threats to the DPS that would require regulatory protections.
For the reasons described above, we conclude that existing regulatory mechanisms are adequate to protect the Upper Missouri River DPS of Arctic grayling. We do not anticipate any changes to the existing regulatory mechanisms; thus we conclude that existing regulatory mechanisms will remain adequate in the future.
Factor E. Other Natural or Manmade Factors Affecting Its Continued Existence
Drought
Drought is a natural occurrence in the interior western United States (see National Drought Mitigation Center 2010). The duration and severity of drought in Montana appears to have increased during the last 50 years, and precipitation has tended to be lower than average in the last 20 years (National Climatic Data Center 2010). Drought can affect fish populations by reducing stream flow volumes. This leads to dewatering and high temperatures that can limit connectivity among spawning, rearing, and sheltering habitats. Drought can also reduce the volume of thermally suitable habitat and increase the frequency of water temperatures above the physiological limits for optimum growth and survival in Arctic grayling. In addition, drought can interact with human-caused stressors (e.g., irrigation withdrawals, riparian habitat degradation) to further reduce stream flows and increase water temperatures.
Reduced stream flows and elevated water temperatures during drought have been most apparent in the Big Hole River system (Magee and Lamothe 2003, pp. 10-14; Magee et al. 2005, pp. 23-25; Rens and Magee 2007, pp. 11-12, 14). In the Big Hole River, evidence for the detrimental effects of drought on Arctic grayling populations is primarily inferential; observed declines in fluvial Arctic grayling and nonnative trout abundances in the Big Hole River coincide with periods of drought (Magee and Lamothe 2003, pp. 22-23, 28) and fish kills (Byorth 1995, pp. 10-11, 31).
Although the response of stream and river habitats to drought is expected to be most pronounced because of the strong seasonality of flows in those habitats, effects in lake environments can occur. For example, both the Upper and Lower Red Rock Lakes are very shallow (Mogen 1996, p. 7). Increased frequency or duration of drought could lead to increased warming in shallower lakes, such as Upper Red Rock Lake. However, the Centennial Valley has many springs sources that could, at least in part, mitigate for increases in water temperature due to increased drought frequency and magnitude. Other potential effects from drought could include a reduction in overall lake depth, which could in turn affect summer or overwintering habitat. Adfluvial populations in high mountain lakes would likely not be affected significantly by drought because air (and thus water) temperatures in these habitats are relatively cool due to the greater distance from sea level at high elevations (∼ a 3.6 °F (6.5 °C) decrease in air temperature for every 3,200 ft. (1 kilometer) above sea level; Physics 2014). In addition, most of these habitats are relatively large bodies of water volumetrically, thus are resistant to warming, given the high specific heat of water (USGS 2014). Further, intact riparian areas in these habitats buffer against water temperature increases in tributaries by blocking incoming solar radiation (Sridhar et al. 2004, entire; Cassie 2006, p. 1393).
Given the climate of the intermountain West, we conclude that drought has been and will continue to be a natural occurrence. We assume that negative effects of drought on Arctic grayling populations, such as reduced connectivity among habitats or increased water temperatures at or above physiological thresholds for growth and survival, are more frequent in stream and river environments and in very shallow lakes relative to larger, deeper lakes. As discussed under Factor A, the implementation of the Big Hole Arctic grayling CCAA is likely to minimize some of the effects of drought in the Big Hole River, by reducing the likelihood that human-influenced actions or outcomes (irrigation withdrawals, destruction of riparian habitats, and fish passage barriers) will interact with the natural effects of drought (reduced stream flows and increased water temperatures). We expect the impact of drought may act at the individual level, but not at the population or DPS level because most grayling populations reside in drought-resistant habitats in high mountain lakes. Some populations will likely be affected by drought, but implemented conservation measures (Big Hole River population) and natural spring sources (Centennial Valley) are expected to minimize the impact. Overall, we conclude that drought has been a past threat when many historical habitats were degraded, but is not a current threat because of the intact nature of most habitats occupied by Arctic grayling in the upper Missouri River basin. Drought is expected to increase in both duration and severity in the future; however, resiliency currently being incorporated into riparian and aquatic habitats through conservation projects will likely buffer the effects of drought. Thus, drought is not expected to pose a threat to the DPS in the future.
Stochastic (Random) Threats, Genetic Diversity and Small Population Size
A principle of conservation biology is that the presence of larger and more productive (resilient) populations can reduce overall extinction risk. To minimize extinction risk due to stochastic (random) threats, life-history diversity should be maintained, populations should not all share common catastrophic risks, and both widespread and spatially close populations are needed (Fausch et al. 2006, p. 23; Allendorf et al. 1997, entire).
The Upper Missouri River DPS of Arctic grayling exists largely as a collection of isolated populations (Peterson and Ardren 2009, entire), with little to no gene flow among populations. While the inability of fish to move between populations limits genetic exchange and demographic support (Hilderbrand 2003, p. 257), large population sizes coupled with adequate number of breeding individuals minimize the effects of isolation. For example, Grebe Lake, a large population, receives no genetic infusion from any other population in the upper Missouri River basin, yet has a very large estimated effective population size (see Table 3, above). Loss of genetic diversity from genetic drift is not a concern for this population, despite it being reproductively isolated.
Abundance among the 20 Arctic grayling populations varies widely (see Table 3, above). Individually, small populations like Ruby River need to maintain enough adults to minimize loss of variability through genetic drift and inbreeding (Rieman and McIntyre 1993, pp. 10-11). The point estimates of the effective number of breeders observed in all populations (where data are available) are above the level at which inbreeding is an immediate concern (Leary 2014, pers. comm.). The Ruby River population exhibits a low effective number of breeders, but contains the second highest genetic diversity among all populations (Leary 2014, unpublished data). Thus, inbreeding depression is probably not a concern for this population in the near future (Leary 2014, pers. comm.).
Effective population size estimates for other Arctic grayling populations vary from 162 to 1,497 (see Table 3, above). There has been considerable debate about what effective population size is adequate to conserve genetic diversity and long-term adaptive potential (see Jamieson and Allendorf 2012 for review, p. 579). However, loss of genetic diversity is typically not an immediate threat even in isolated populations with an N e>100 (Palstra and Ruzzante 2008, p. 3441), but rather is a symptom of deterministic processes acting on the population (Jamieson and Allendorf 2012, p. 580). In other words, loss of genetic diversity due to small effective population size typically does not drive species to extinction (Jamieson and Allendorf 2012, entire); other processes, such as habitat degradation, have a more immediate and greater impact on species persistence (Jamieson and Allendorf 2012). We acknowledge that loss of genetic diversity can occur in small populations; however, in this case, it appears that there are adequate numbers of breeding adults to minimize loss of genetic diversity. Thus, we conclude that loss of genetic diversity is not a threat at the DPS level.
Conservation of life-history diversity is important to the persistence of species confronted by habitat change and environmental perturbations (Beechie et al. 2006, entire). Therefore, the reintroductions of fluvial Arctic grayling into the upper Ruby River that have occurred provide redundancy of the fluvial ecotype. The number of breeding individuals in the Ruby River population has increased over the last 3 years (Leary 2014, unpublished data). Thus, there is now a viable replicate of the fluvial ecotype.
Populations of Arctic grayling in the Upper Missouri River DPS are for the most part widely separated from one another, occupying 7 of 10 historically occupied watersheds (see Table 3, above). Thus, risk of extirpation by a rare, high-magnitude environmental disturbance (i.e., catastrophe) is relatively low. In addition, multiple spawning locations exist for 11 of the 20 populations in the Upper Missouri River DPS. The 11 populations with access to multiple spawning tributaries include all the largest populations in terms of abundance, except Mussigbrod Lake (see Table 3). Abundance and number of breeding individuals is adequate in most populations to sustain moderate to high levels of genetic diversity currently observed. Based on this information, we conclude that stochastic processes are not a threat to the Upper Missouri River DPS of Arctic grayling and are not expected to be in the future.
Summary of Factor E
Overall, we conclude that the Upper Missouri River DPS of Arctic grayling has faced historical threats from drought, loss of genetic diversity, and small population size. However, the DPS currently exists as multiple, isolated populations across a representative portion of its historical range. While reproductive isolation can lead to detrimental genetic effects, the current size of most Arctic grayling populations, trends in effective population size, and number of breeders suggest these effects will be minimal. Redundancies within and among populations are present: Multiple spawning tributaries, geographic separation, life-history replication. Given this information, we conclude the redundant nature of multiple resilient populations across a representative portion of the species’ historical range minimizes the impacts of drought, low abundance, reduced genetic diversity, and lack of a fluvial ecotype replicate. Thus, these are not current threats, and are not expected to be threats in the future.
Cumulative Effects From Factors A Through E
We limit our discussion of cumulative effects from Factors A through E to interactions involving climate change. Our rationale for this is that climate change has the highest level of uncertainty among other factors considered, and likely has the most potential to affect Arctic grayling populations when interacting with other factors.
Climate Change and Nonnative Species Interactions
Changes in water temperature due to climate change may influence the distribution of nonnative trout species (Rahel and Olden 2008, p. 524) and the outcome of competitive interactions between those species and Arctic grayling. Brown trout are generally considered to be more tolerant of warm water than many salmonid species common in western North America (Coutant 1999, pp. 52-53; Selong et al. 2001, p. 1032), and higher water temperatures may favor brown trout where they compete against salmonids with lower thermal tolerances (Rahel and Olden 2008, p. 524). Recently, observed increases in the abundance and distribution of brown trout in the upper reaches of the Big Hole River (MFWP 2013, unpublished data) may be consistent with the hypothesis that stream warming is facilitating encroachment. However, the effect of increased abundance and distribution of brown trout on Arctic grayling in the Big Hole River is unknown.
Currently, brown trout are at relatively low densities (<20 fish/mile) in the upper Big Hole River, where Arctic grayling densities are highest (MFWP 2013e, unpublished data). At densities of 100 brown trout per mile (a plausible future scenario), Arctic grayling experts predicted a 5 percent reduction in Arctic grayling recruitment in the Big Hole River, due to competition and predation (SSA 2014, p. 2). Given that natural mortality of salmonid fry is typically high (>90 percent) (Kruse 1959, pp. 329, 333; Bradford 1995, p. 1330), the predicted reductions in Arctic grayling recruitment by current and future densities of brown trout in the Big Hole River will likely not impact Arctic grayling at the population level. Thus, the potential cumulative effect of climate change and nonnative species interactions is not a current or future threat for the Upper Missouri River DPS of Arctic grayling.
Climate Change and Dewatering
Synergistic interactions are possible between effects of climate change and effects of other potential stressors such as dewatering. Increases in temperature and changes in precipitation are likely to affect the availability of water in the West. However, it is difficult to project how climate change will affect water availability because increased air and water temperatures may be accompanied and tempered by more frequent precipitation events. Uncertainty about how different temperature and precipitation scenarios could affect water availability make projecting possible synergistic effects of climate change on the Arctic grayling too speculative at this time.
Summary
Recent genetic analyses have concluded that many of the introduced populations of Arctic grayling in the upper Missouri River basin contain moderate to high levels of genetic diversity and that these populations were created from local sources within the basin. These introduced populations currently occur within the confines of the upper Missouri River basin and occupy high quality habitats on Federal land, the same places the Service would look to for long-term conservation of the species, if needed. As such, these populations and their future adaptive potential have conservation value and are included in the Upper Missouri River DPS of Arctic grayling.
Currently, we recognize 20 populations of Arctic grayling in the Upper Missouri River DPS, 18 of which occur on Federal land. Adequate regulatory mechanisms exist to ensure the conservation of habitat on Federal land for these populations. Historical habitat degradation on private land has affected the Big Hole River population; however, habitat conditions have been improving since the implementation of the Big Hole CCAA in 2006. Conservation actions associated with the Big Hole CCAA and SHCP have reduced water temperatures in tributaries, increased instream flows in tributaries and the mainstem Big Hole River, connected almost all core habitat for Arctic grayling, and improved riparian health. Arctic grayling have responded favorably to these improvements because abundance and distribution have increased throughout the upper Big Hole River, and number of breeding adults has increased by a factor of at least 5 since 2006. The Service is encouraged by the successful track record of conservation actions implemented under the Big Hole CCAA and SHCP over the past 7 years.
Riparian restoration efforts in the Big Hole River and Centennial Valley are ongoing and will continue to be key in mitigating the anticipated effects of drought and climate change. Increased shading of tributaries and decreased width-to-depth ratios in stream channels can effectively minimize effects from increasing air temperatures and drought. In addition, these changes to habitat can alter predation and competition potential where both nonnative species and Arctic grayling coexist, as they have for over 100 years in some populations.
We acknowledge the uncertainty regarding the current status of the Ennis Reservoir/Madison River population and probable declining trend in abundance. The factors influencing the current demographics of this population are unclear. However, we are encouraged by the recent FERC relicensing agreement precluding reservoir drawdowns that likely affected this population and its habitat in the past.
In conclusion, we find viable populations of both ecotypes present in the DPS, the majority of which occur on Federal land and are protected by Federal land management measures. Numbers of breeding adults are currently increasing in both strictly fluvial populations and in the Centennial Valley. High-quality habitat is present for most populations or is improving where it is not optimal (e.g., Big Hole River). Health of riparian areas is trending upward and will be key to minimizing effects of climate change and drought. All Arctic grayling populations are genetically diverse, are of Montana-origin, and occur in 7 of 10 historically occupied watersheds.
In 2010, we identified multiple threats as acting on the Upper Missouri River DPS of Arctic grayling. At that time, we determined that habitat-related threats included habitat fragmentation, dewatering, thermal stress, entrainment, riparian habitat loss, and effects from climate change. Since 2010, we have 4 additional years of monitoring data and have gained new insight. It is now apparent that these threats are being effectively mitigated on private land (Big Hole River) by conservation actions under the Big Hole CCAA and do not appear to be present or acting at a level to warrant concern on most of the adfluvial populations. Almost all (98 percent) of Arctic grayling core habitat in the Big Hole River is now connected. Recent riparian restoration activities have appreciably reduced water temperatures and improved riparian habitat in tributaries to the Big Hole River and are expected to buffer the effects of climate change. Entrainment of Arctic grayling into irrigation canals in the Big Hole system is low, with no documented entrainment occurring since 2010. Habitats on Federal land are largely intact and these populations are not subject to many of the stressors historically identified for other populations because no irrigation diversions are present, habitats are primarily high-elevation lakes that have cool water temperatures, and riparian areas are largely intact.
In 2010, another threat identified as acting on the Upper Missouri River DPS of Arctic grayling was the presence of nonnative trout. We considered nonnative trout a threat at that time because we were aware of several instances where Arctic grayling declines had occurred following nonnative trout introductions. Currently, we have a better understanding of the interactions between nonnative trout and Arctic grayling. Our review of these interactions and case histories suggests that habitat degradation, concurrent with nonnative trout introductions, likely contributed to historical declines in Arctic grayling in those instances. Further, it appears the effect of nonnative trout on Arctic grayling are likely habitat-mediated; nonnative trout affect Arctic grayling disproportionately when habitat conditions are degraded, but both Arctic grayling and nonnatives can coexist at viable levels when habitat conditions are improved. The primary evidence supporting this assertion is the increasing abundance and distribution of both Arctic grayling and nonnatives in the Big Hole River (brown trout) and Centennial Valley (Yellowstone cutthroat trout before suppression began). Another line of evidence to support this assertion is observed spatial segregation between nonnatives and Arctic grayling in the core Arctic grayling areas in the Big Hole River, especially spawning and rearing areas (SSA 2014). In addition, Arctic grayling in adfluvial habitats have maintained stable or increasing population levels in the presence of brook, rainbow, and Yellowstone cutthroat trout for over 100 years in many instances in the upper Missouri River basin, where habitat degradation has not occurred or been extensive.
In 2010, we stated that existing regulatory mechanisms were inadequate to protect the Upper Missouri River DPS of Arctic grayling. The primary reason for this assertion was that Arctic grayling populations were reported as declining; thus existing regulatory mechanisms were believed to be inadequate because they had failed to halt or reverse this decline. Currently, we have updated information indicating that 19 of 20 populations of Arctic grayling are either stable or increasing. Existing regulatory mechanisms have precluded riparian habitat destruction on Federal lands or mandated restoration of impaired areas and are expected to provide similar protections in the future. Given the updated information, we now believe these regulatory mechanisms are adequate.
In 2010, we identified reduced genetic diversity, low abundance, random events, drought, and lack of a fluvial replicate as threats to the Upper Missouri River DPS of Arctic grayling. Updated genetic information that was not available in 2010 indicates moderate to high levels of genetic diversity within most Arctic grayling populations in the DPS. Further, abundance estimates derived from this updated genetic information indicate higher Arctic grayling abundances than previously thought. Adequate redundancy exists within the DPS to minimize the effects of random events and drought; lake habitats occupied by most Arctic grayling populations are drought-resistant. Lastly, a viable fluvial replicate now exists (Ruby River), with 5 years of natural reproduction documented and an increasing number of breeding adults.
Finding Back to Top
As required by the Act, we considered the five factors in assessing whether the Upper Missouri River DPS of Arctic grayling is endangered or threatened throughout all of its range. We examined the best scientific and commercial information available regarding the present and future threats faced by the Upper Missouri River DPS of Arctic grayling. We reviewed the petition, information available in our files and other available published and unpublished information, including information submitted by the public, and we consulted with recognized Arctic grayling experts and other Federal and State agencies. Habitat-related threats previously identified, including habitat fragmentation, dewatering, thermal stress, entrainment, riparian habitat loss, and effects from climate change, have been sufficiently ameliorated and the information indicates that 19 of 20 populations of Arctic grayling are either stable or increasing. On the basis of the best scientific and commercial information available and the analysis provided above, we find that the magnitude and imminence of threats do not indicate that the Upper Missouri River DPS of Arctic grayling is in danger of extinction (endangered), or likely to become endangered within the foreseeable future (threatened), throughout its range. Therefore, we find that listing the Upper Missouri River DPS throughout its range as a threatened or an endangered species is not warranted at this time.
Significant Portion of the Range Back to Top
Under the Act and our implementing regulations, a species may warrant listing if it is an endangered or a threatened species throughout all or a significant portion of its range. The Act defines “endangered species” as any species which is “in danger of extinction throughout all or a significant portion of its range,” and “threatened species” as any species which is “likely to become an endangered species within the foreseeable future throughout all or a significant portion of its range.” The term “species” includes “any subspecies of fish or wildlife or plants, and any distinct population segment [DPS] of any species of vertebrate fish or wildlife which interbreeds when mature.” On July 1, 2014, we published a final policy interpreting the phrase “Significant Portion of its Range” (SPR) (79 FR 37578). The final policy states that (1) if a species is found to be an endangered or a threatened species throughout a significant portion of its range, the entire species is listed as an endangered or a threatened species, respectively, and the Act’s protections apply to all individuals of the species wherever found; (2) a portion of the range of a species is “significant” if the species is not currently an endangered or a threatened species throughout all of its range, but the portion’s contribution to the viability of the species is so important that, without the members in that portion, the species would be in danger of extinction, or likely to become so in the foreseeable future, throughout all of its range; (3) the range of a species is considered to be the general geographical area within which that species can be found at the time FWS or NMFS makes any particular status determination; and (4) if a vertebrate species is an endangered or a threatened species throughout an SPR, and the population in that significant portion is a valid DPS, we will list the DPS rather than the entire taxonomic species or subspecies.
The SPR policy is applied to all status determinations, including analyses for the purposes of making listing, delisting, and reclassification determinations. The procedure for analyzing whether any portion is an SPR is similar, regardless of the type of status determination we are making. The first step in our analysis of the status of a species is to determine its status throughout all of its range. If we determine that the species is in danger of extinction, or likely to become so in the foreseeable future, throughout all of its range, we list the species as an endangered (or threatened) species and no SPR analysis will be required. If the species is neither an endangered nor a threatened species throughout all of its range, we determine whether the species is an endangered or a threatened species throughout a significant portion of its range. If it is, we list the species as an endangered or a threatened species, respectively; if it is not, we conclude that listing the species is not warranted.
When we conduct an SPR analysis, we first identify any portions of the species’ range that warrant further consideration. The range of a species can theoretically be divided into portions in an infinite number of ways. However, there is no purpose to analyzing portions of the range that are not reasonably likely to be significant and either an endangered or a threatened species. To identify only those portions that warrant further consideration, we determine whether there is substantial information indicating that (1) the portions may be significant and (2) the species may be in danger of extinction in those portions or likely to become so within the foreseeable future. We emphasize that answering these questions in the affirmative is not a determination that the species is an endangered or a threatened species throughout a significant portion of its range—rather, it is a step in determining whether a more detailed analysis of the issue is required. In practice, a key part of this analysis is whether the threats are geographically concentrated in some way. If the threats to the species are affecting it uniformly throughout its range, no portion is likely to warrant further consideration. Moreover, if any concentration of threats apply only to portions of the range that clearly do not meet the biologically based definition of “significant” (i.e., the loss of that portion clearly would not be expected to increase the vulnerability to extinction of the entire species), those portions will not warrant further consideration.
If we identify any portions that may be both (1) significant and (2) endangered or threatened, we engage in a more detailed analysis to determine whether these standards are indeed met. The identification of an SPR does not create a presumption, prejudgment, or other determination as to whether the species in that identified SPR is an endangered or a threatened species. We must go through a separate analysis to determine whether the species is an endangered or a threatened species in the SPR. To determine whether a species is an endangered or a threatened species throughout an SPR, we will use the same standards and methodology that we use to determine if a species is an endangered or a threatened species throughout its range.
Depending on the biology of the species, its range, and the threats it faces, it may be more efficient to address the “significant” question first, or the status question first. Thus, if we determine that a portion of the range is not “significant,” we do not need to determine whether the species is an endangered or a threatened species there; if we determine that the species is not an endangered or a threatened species in a portion of its range, we do not need to determine if that portion is “significant.”
We evaluated the current range of the Upper Missouri River DPS of Arctic grayling to determine if there is any apparent geographic concentration of potential threats. We examined potential threats from curtailment of range, dams, habitat fragmentation, dewatering and thermal stress, entrainment, riparian habitat loss, sediment, exploitation, disease and competition/predation, drought, climate change, stochastic events, reduced genetic diversity, low abundance, and lack of a fluvial ecotype replicate. The type and magnitude of stressors acting on the Arctic grayling populations in the DPS are varied.
Currently, nineteen of the twenty Arctic grayling populations in the DPS are stable or increasing in abundance. Given this trend, we conclude that there is no concentration of threats acting on these nineteen populations because these populations are able to maintain viability, despite some stressors acting at the individual level on some of these populations. However, we acknowledge the probable declining population trend in the Ennis Reservoir/Madison River population. It is unclear what factor or combination of factors is contributing to this decline. Nonnative trout abundance is highest in the Madison River, relative to all other systems occupied by nonnative trout and Arctic grayling, and this factor may be contributing to the decline of Arctic grayling in Ennis Reservoir/Madison River.
Given the probable decline of Arctic grayling in Ennis Reservoir/Madison River, we analyzed the potential significance of this population to the overall Upper Missouri River DPS of Arctic grayling. To do this analysis, we evaluated whether the Ennis Reservoir/Madison River population’s contribution to the viability of the DPS is so important that, without the members in this portion, the DPS would be in danger of extinction, or likely to become so in the foreseeable future, throughout all of its range. The Ennis Reservoir/Madison River population occupies a small portion of the range within the DPS and represents only 1 of 20 populations in the overall DPS. We conclude that the DPS would still be viable if the Ennis Reservoir/Madison River population were extirpated because adequate redundancy (3 other fluvial or partially fluvial and 16 other adfluvial populations) of Arctic grayling populations would still exist. In addition, representation of resilient populations would remain in the Madison drainage (Grebe Lake population) and rangewide in 7 of 10 historically occupied watersheds in the Upper Missouri River basin. Further, resiliency of the DPS would not be compromised by the loss of the Ennis Reservoir/Madison River population because all remaining Arctic grayling populations are widespread and viable. Therefore, in the hypothetical absence of the Ennis Reservoir/Madison River population, the remainder of the Upper Missouri River DPS of Arctic grayling would not meet the definition of threatened or endangered under the Act. For the reasons stated above, the Ennis Reservoir/Madison River population does not meet the definition of “significant” for the purposes of this SPR analysis.
In conclusion, we find no concentration of stressors acting on nineteen of twenty Arctic grayling populations in the DPS. The Ennis Reservoir/Madison River population does appear to have a stressor or combination of stressors acting at the population level. However, further analysis indicates that the Ennis Reservoir/Madison River does not meet the definition of “significant” in our SPR policy because adequate redundancy, representation, and resiliency would still exist within the DPS if the Ennis Reservoir/Madison River population were extirpated. Thus, the remainder of the Upper Missouri River DPS of Arctic grayling would not meet the definition of threatened or endangered. Therefore, we find that there is not a significant portion of the range of the Upper Missouri River DPS of Arctic grayling that warrants listing.
Our review of the best available scientific and commercial information indicates that the Upper Missouri River DPS of Arctic grayling is not in danger of extinction (endangered), nor likely to become endangered within the foreseeable future (threatened), throughout all or a significant portion of its range. Therefore, we find that listing the Upper Missouri River DPS of Arctic grayling as an endangered or threatened species under the Act is not warranted at this time.
We request that you submit any new information concerning the status of, or threats to, the Upper Missouri River DPS of Arctic grayling to our Montana Ecological Services Office (see ADDRESSES) whenever it becomes available. New information will help us monitor the Upper Missouri River DPS of Arctic grayling and encourage its conservation. If an emergency situation develops for the Upper Missouri River DPS of Arctic grayling, we will act to provide immediate protection.
References Cited Back to Top
A complete list of references cited is available on the Internet at http://www.regulations.gov and upon request from the Montana Ecological Services Office (see ADDRESSES).
Authors Back to Top
The primary authors of this document are the staff members of the Montana Ecological Services Office.
Authority Back to Top
The authority for this section is section 4 of the Endangered Species Act of 1973, as amended (16 U.S.C. 1531 et seq.).
Dated: August 6, 2014.
David Cottingham,
Acting Director, Fish and Wildlife Service.
[FR Doc. 2014-19353 Filed 8-19-14; 4:15 pm]
BILLING CODE 4310-55-P